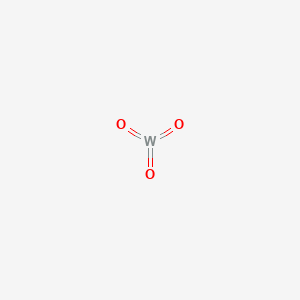
Tungsten trioxide
Overview
Description
Tungsten trioxide, also known as tungsten(VI) oxide or tungstic anhydride, is a chemical compound composed of oxygen and tungsten with the formula WO₃. It is a light yellow crystalline solid that occurs naturally in the form of hydrates, such as tungstite (WO₃·H₂O), meymacite (WO₃·2H₂O), and hydrotungstite. These minerals are rare secondary tungsten minerals .
Mechanism of Action
Target of Action
Tungsten trioxide (WO3) is a versatile compound with a wide range of applications, including its use as a catalyst, sensor, and in electrochromic devices . The primary targets of WO3 are the biomolecules or pathways essential for bacterial survival . By simultaneously attacking these targets, WO3 prevents the development of resistance against a single target .
Mode of Action
WO3 interacts with its targets primarily through its unique properties and mechanisms of action. These include its ability to generate reactive oxygen species and induce photothermal effects, leading to bacterial cell death . The WO3 nanoparticles can effectively inhibit bacterial growth and exert bactericidal effects . Their ability to induce oxidative stress, disrupt cell membranes, and interfere with essential cellular processes collectively contribute to their antibacterial efficacy .
Biochemical Pathways
The biochemical pathways affected by WO3 are primarily those essential for bacterial survival. By attacking multiple biomolecules or pathways, WO3 nanoparticles prevent the development of resistance against a single target . This multi-target approach enhances the effectiveness of WO3 as an antibacterial agent.
Pharmacokinetics
It’s important to note that the effectiveness of wo3 nanoparticles as antibacterial agents can be influenced by their potential toxicity and compatibility with human cells .
Result of Action
The result of WO3’s action is the effective inhibition of bacterial growth and the induction of bacterial cell death . This is achieved through the generation of reactive oxygen species, disruption of cell membranes, and interference with essential cellular processes .
Action Environment
The action of WO3 can be influenced by various environmental factors. For instance, the photocatalytic activity of WO3 can be enhanced under visible light . Additionally, the synthesis method and subsequent post-synthesis treatments can create a wide variety of different morphologies, phases, exposed facets, and defect structures, all of which must be carefully considered for the chosen application .
Biochemical Analysis
Biochemical Properties
Tungsten trioxide plays a crucial role in biochemical reactions, particularly in catalysis and sensing. It interacts with various enzymes, proteins, and other biomolecules. For instance, this compound can act as a catalyst in oxidation-reduction reactions, interacting with enzymes such as oxidases and reductases. These interactions often involve the transfer of electrons, which facilitates the catalytic process. Additionally, this compound can bind to proteins and other biomolecules, altering their conformation and activity, thereby influencing biochemical pathways .
Cellular Effects
This compound has been shown to affect various types of cells and cellular processes. It can influence cell function by modulating cell signaling pathways, gene expression, and cellular metabolism. For example, this compound can activate or inhibit specific signaling pathways, leading to changes in gene expression and metabolic activity. These effects can result in altered cell proliferation, differentiation, and apoptosis, depending on the concentration and exposure duration .
Molecular Mechanism
The molecular mechanism of this compound involves its interaction with biomolecules at the molecular level. This compound can bind to enzymes, inhibiting or activating their activity. This binding can lead to changes in the enzyme’s conformation and function, affecting biochemical pathways. Additionally, this compound can influence gene expression by interacting with transcription factors and other regulatory proteins, leading to changes in the expression of specific genes .
Temporal Effects in Laboratory Settings
In laboratory settings, the effects of this compound can change over time. The stability and degradation of this compound are critical factors that influence its long-term effects on cellular function. Studies have shown that this compound can degrade over time, leading to changes in its catalytic activity and interactions with biomolecules. Long-term exposure to this compound can result in cumulative effects on cellular function, including alterations in cell signaling, gene expression, and metabolism .
Dosage Effects in Animal Models
The effects of this compound vary with different dosages in animal models. At low doses, this compound may have minimal or beneficial effects, such as enhancing catalytic activity or modulating cell signaling pathways. At high doses, this compound can exhibit toxic or adverse effects, including oxidative stress, inflammation, and cell death. These threshold effects highlight the importance of dosage in determining the safety and efficacy of this compound in biological systems .
Metabolic Pathways
This compound is involved in various metabolic pathways, interacting with enzymes and cofactors. It can influence metabolic flux and metabolite levels by modulating the activity of key enzymes in metabolic pathways. For example, this compound can affect the activity of enzymes involved in oxidative phosphorylation, glycolysis, and the tricarboxylic acid cycle, leading to changes in energy production and metabolic homeostasis .
Transport and Distribution
Within cells and tissues, this compound is transported and distributed through various mechanisms. It can interact with transporters and binding proteins, influencing its localization and accumulation. This compound can be taken up by cells through endocytosis or passive diffusion, and it can accumulate in specific cellular compartments, such as the cytoplasm or mitochondria. These interactions can affect the compound’s activity and function within the cell .
Subcellular Localization
The subcellular localization of this compound is critical for its activity and function. This compound can be directed to specific compartments or organelles through targeting signals or post-translational modifications. For example, this compound can localize to the mitochondria, where it can influence mitochondrial function and energy production. Additionally, this compound can be targeted to the nucleus, where it can interact with transcription factors and other regulatory proteins to modulate gene expression .
Preparation Methods
Synthetic Routes and Reaction Conditions: Tungsten trioxide can be synthesized through various methods, including:
Thermal Decomposition: Ammonium paratungstate ((NH₄)₁₀[H₂W₁₂O₄₂]·4H₂O) is thermally decomposed to produce this compound. The decomposition occurs at temperatures around 600°C.
Precipitation Method: this compound can be prepared by precipitating tungstic acid from an aqueous solution of sodium tungstate (Na₂WO₄) using an acid, followed by calcination at high temperatures.
Industrial Production Methods: In industrial settings, this compound is typically produced by:
Chemical Reactions Analysis
Tungsten trioxide undergoes various types of chemical reactions, including:
Oxidation: this compound can be further oxidized to form higher oxidation states of tungsten, such as tungsten pentoxide (WO₅).
Reduction: this compound can be reduced to lower oxidation states, such as tungsten dioxide (WO₂), using reducing agents like hydrogen or carbon monoxide.
Substitution: this compound can react with halogens to form tungsten halides, such as tungsten hexachloride (WCl₆).
Common Reagents and Conditions:
Oxidation: Oxygen or ozone at elevated temperatures.
Reduction: Hydrogen gas or carbon monoxide at high temperatures.
Substitution: Halogens like chlorine or bromine at elevated temperatures.
Major Products Formed:
Oxidation: Tungsten pentoxide (WO₅).
Reduction: Tungsten dioxide (WO₂).
Substitution: Tungsten hexachloride (WCl₆).
Scientific Research Applications
Tungsten trioxide has a wide range of scientific research applications, including:
Comparison with Similar Compounds
- Molybdenum trioxide (MoO₃)
- Chromium trioxide (CrO₃)
- Vanadium pentoxide (V₂O₅)
- Tungsten dioxide (WO₂)
- Tungsten pentoxide (WO₅)
Tungsten trioxide stands out due to its unique combination of high stability, versatile catalytic properties, and wide range of applications in various fields.
Properties
IUPAC Name |
trioxotungsten | |
---|---|---|
Source | PubChem | |
URL | https://pubchem.ncbi.nlm.nih.gov | |
Description | Data deposited in or computed by PubChem | |
InChI |
InChI=1S/3O.W | |
Source | PubChem | |
URL | https://pubchem.ncbi.nlm.nih.gov | |
Description | Data deposited in or computed by PubChem | |
InChI Key |
ZNOKGRXACCSDPY-UHFFFAOYSA-N | |
Source | PubChem | |
URL | https://pubchem.ncbi.nlm.nih.gov | |
Description | Data deposited in or computed by PubChem | |
Canonical SMILES |
O=[W](=O)=O | |
Source | PubChem | |
URL | https://pubchem.ncbi.nlm.nih.gov | |
Description | Data deposited in or computed by PubChem | |
Molecular Formula |
WO3, O3W | |
Record name | tungsten(VI) oxide | |
Source | Wikipedia | |
URL | https://en.wikipedia.org/wiki/Tungsten(VI)_oxide | |
Description | Chemical information link to Wikipedia. | |
Source | PubChem | |
URL | https://pubchem.ncbi.nlm.nih.gov | |
Description | Data deposited in or computed by PubChem | |
DSSTOX Substance ID |
DTXSID7032262 | |
Record name | Tungsten trioxide | |
Source | EPA DSSTox | |
URL | https://comptox.epa.gov/dashboard/DTXSID7032262 | |
Description | DSSTox provides a high quality public chemistry resource for supporting improved predictive toxicology. | |
Molecular Weight |
231.84 g/mol | |
Source | PubChem | |
URL | https://pubchem.ncbi.nlm.nih.gov | |
Description | Data deposited in or computed by PubChem | |
Physical Description |
Dry Powder; Dry Powder, Other Solid, NKRA; Dry Powder, Pellets or Large Crystals, Other Solid; Other Solid; Pellets or Large Crystals, Canary yellow solid; [Merck Index] Blue or brown odorless powder; [MSDSonline] Insoluble in water; [Hawley] | |
Record name | Tungsten oxide (WO3) | |
Source | EPA Chemicals under the TSCA | |
URL | https://www.epa.gov/chemicals-under-tsca | |
Description | EPA Chemicals under the Toxic Substances Control Act (TSCA) collection contains information on chemicals and their regulations under TSCA, including non-confidential content from the TSCA Chemical Substance Inventory and Chemical Data Reporting. | |
Record name | Tungsten trioxide | |
Source | Haz-Map, Information on Hazardous Chemicals and Occupational Diseases | |
URL | https://haz-map.com/Agents/7672 | |
Description | Haz-Map® is an occupational health database designed for health and safety professionals and for consumers seeking information about the adverse effects of workplace exposures to chemical and biological agents. | |
Explanation | Copyright (c) 2022 Haz-Map(R). All rights reserved. Unless otherwise indicated, all materials from Haz-Map are copyrighted by Haz-Map(R). No part of these materials, either text or image may be used for any purpose other than for personal use. Therefore, reproduction, modification, storage in a retrieval system or retransmission, in any form or by any means, electronic, mechanical or otherwise, for reasons other than personal use, is strictly prohibited without prior written permission. | |
Solubility |
Insol in water; sol in caustic alkalies; very slightly sol in acids, SLIGHTLY SOL IN HYDROFLUORIC ACID | |
Record name | TUNGSTEN TRIOXIDE | |
Source | Hazardous Substances Data Bank (HSDB) | |
URL | https://pubchem.ncbi.nlm.nih.gov/source/hsdb/5800 | |
Description | The Hazardous Substances Data Bank (HSDB) is a toxicology database that focuses on the toxicology of potentially hazardous chemicals. It provides information on human exposure, industrial hygiene, emergency handling procedures, environmental fate, regulatory requirements, nanomaterials, and related areas. The information in HSDB has been assessed by a Scientific Review Panel. | |
Density |
7.2 | |
Record name | TUNGSTEN TRIOXIDE | |
Source | Hazardous Substances Data Bank (HSDB) | |
URL | https://pubchem.ncbi.nlm.nih.gov/source/hsdb/5800 | |
Description | The Hazardous Substances Data Bank (HSDB) is a toxicology database that focuses on the toxicology of potentially hazardous chemicals. It provides information on human exposure, industrial hygiene, emergency handling procedures, environmental fate, regulatory requirements, nanomaterials, and related areas. The information in HSDB has been assessed by a Scientific Review Panel. | |
Color/Form |
Canary yellow, heavy powder; dark orange when heated, regaining the original color on cooling, Yellow, rhombic crystals, or yellow-orange powder | |
CAS No. |
1314-35-8 | |
Record name | Tungsten oxide (WO3) | |
Source | CAS Common Chemistry | |
URL | https://commonchemistry.cas.org/detail?cas_rn=1314-35-8 | |
Description | CAS Common Chemistry is an open community resource for accessing chemical information. Nearly 500,000 chemical substances from CAS REGISTRY cover areas of community interest, including common and frequently regulated chemicals, and those relevant to high school and undergraduate chemistry classes. This chemical information, curated by our expert scientists, is provided in alignment with our mission as a division of the American Chemical Society. | |
Explanation | The data from CAS Common Chemistry is provided under a CC-BY-NC 4.0 license, unless otherwise stated. | |
Record name | Tungsten trioxide | |
Source | ChemIDplus | |
URL | https://pubchem.ncbi.nlm.nih.gov/substance/?source=chemidplus&sourceid=0001314358 | |
Description | ChemIDplus is a free, web search system that provides access to the structure and nomenclature authority files used for the identification of chemical substances cited in National Library of Medicine (NLM) databases, including the TOXNET system. | |
Record name | Tungsten oxide (WO3) | |
Source | EPA Chemicals under the TSCA | |
URL | https://www.epa.gov/chemicals-under-tsca | |
Description | EPA Chemicals under the Toxic Substances Control Act (TSCA) collection contains information on chemicals and their regulations under TSCA, including non-confidential content from the TSCA Chemical Substance Inventory and Chemical Data Reporting. | |
Record name | Tungsten trioxide | |
Source | EPA DSSTox | |
URL | https://comptox.epa.gov/dashboard/DTXSID7032262 | |
Description | DSSTox provides a high quality public chemistry resource for supporting improved predictive toxicology. | |
Record name | Tungsten trioxide | |
Source | European Chemicals Agency (ECHA) | |
URL | https://echa.europa.eu/substance-information/-/substanceinfo/100.013.848 | |
Description | The European Chemicals Agency (ECHA) is an agency of the European Union which is the driving force among regulatory authorities in implementing the EU's groundbreaking chemicals legislation for the benefit of human health and the environment as well as for innovation and competitiveness. | |
Explanation | Use of the information, documents and data from the ECHA website is subject to the terms and conditions of this Legal Notice, and subject to other binding limitations provided for under applicable law, the information, documents and data made available on the ECHA website may be reproduced, distributed and/or used, totally or in part, for non-commercial purposes provided that ECHA is acknowledged as the source: "Source: European Chemicals Agency, http://echa.europa.eu/". Such acknowledgement must be included in each copy of the material. ECHA permits and encourages organisations and individuals to create links to the ECHA website under the following cumulative conditions: Links can only be made to webpages that provide a link to the Legal Notice page. | |
Record name | TUNGSTEN TRIOXIDE | |
Source | Hazardous Substances Data Bank (HSDB) | |
URL | https://pubchem.ncbi.nlm.nih.gov/source/hsdb/5800 | |
Description | The Hazardous Substances Data Bank (HSDB) is a toxicology database that focuses on the toxicology of potentially hazardous chemicals. It provides information on human exposure, industrial hygiene, emergency handling procedures, environmental fate, regulatory requirements, nanomaterials, and related areas. The information in HSDB has been assessed by a Scientific Review Panel. | |
Melting Point |
1472 °C | |
Record name | TUNGSTEN TRIOXIDE | |
Source | Hazardous Substances Data Bank (HSDB) | |
URL | https://pubchem.ncbi.nlm.nih.gov/source/hsdb/5800 | |
Description | The Hazardous Substances Data Bank (HSDB) is a toxicology database that focuses on the toxicology of potentially hazardous chemicals. It provides information on human exposure, industrial hygiene, emergency handling procedures, environmental fate, regulatory requirements, nanomaterials, and related areas. The information in HSDB has been assessed by a Scientific Review Panel. | |
Q1: What is the molecular formula and weight of tungsten trioxide?
A1: The molecular formula for this compound is WO3. Its molecular weight is 231.85 g/mol.
Q2: What crystal structures can this compound adopt?
A2: this compound can exist in various polymorphs depending on the temperature. The most common forms include monoclinic, tetragonal, orthorhombic, and cubic structures. []
Q3: What does Density Functional Theory (DFT) reveal about this compound's electronic structure?
A3: DFT calculations indicate that WO3, regardless of its crystal structure, is a semiconductor with zero total magnetization. Interestingly, its direct band gap falls within the visible light wavelength range, making it promising for optical applications. []
Q4: How does the electronic polarizability of oxygen in WO3 compare to other compounds?
A4: The electronic polarizability of the O2- ion in this compound is significantly larger than in other related compounds, contributing approximately 87% to the total polarization of WO3. []
Q5: How does the presence of black phosphorus impact the stability of bismuth oxysulfide/tungsten trioxide heterojunctions?
A5: Studies using X-ray diffraction show that incorporating black phosphorus into bismuth oxysulfide/tungsten trioxide heterojunction composites enhances the structural stability of the composite. []
Q6: How does the morphology of this compound affect its performance in photoelectrochemical applications?
A7: The specific morphology of WO3 is crucial for photoelectrochemical applications like water splitting. Low-dimensional structures, such as nanorods and nanoflakes, provide direct pathways for photogenerated carriers and exhibit reduced grain boundaries, leading to superior charge transport efficiency compared to bulk structures. []
Q7: Can this compound be used for organic dye-sensitized solar cells (DSSCs)?
A8: Yes, WO3 nanoparticles can be used as electrodes in DSSCs. Research has demonstrated the fabrication of DSSCs using WO3 nanoparticles synthesized through thermal oxidation and coated onto substrates via electrophoretic deposition. These devices, using henna as a natural dye, showed promising current density–voltage and spectral photocurrent characteristics. []
Q8: What are some methods for preparing this compound nanoparticles?
A8: this compound nanoparticles can be synthesized using various methods, including:
- Sol-gel method: This versatile method involves the hydrolysis and condensation of a tungsten precursor, such as tungsten hexachloride (WCl6), to form a gel that is then dried and calcined to obtain WO3 nanoparticles. [, , , , ]
- Hydrothermal method: This method utilizes high pressure and temperature in an aqueous solution to promote the crystallization and growth of WO3 nanoparticles from a suitable precursor, such as ammonium paratungstate. [, , ]
- Ultrasonic-chemical precipitation: This technique combines chemical precipitation with ultrasonic irradiation to control the particle size and morphology of WO3 nanoparticles. []
- Thermal oxidation: This method involves heating metallic tungsten in an oxygen atmosphere to produce WO3 nanoparticles, often resulting in materials with oxygen deficiencies. []
- Flame assisted spray pyrolysis: This method involves atomizing a precursor solution into a flame, where the high temperatures initiate pyrolysis and oxidation reactions, leading to the formation of WO3 nanoparticles. []
- Template synthesis: This approach utilizes porous materials, such as anodic aluminum oxide (AAO), as templates to guide the growth of WO3 nanostructures with specific morphologies, such as nanotubes. []
- Biopolymer templating: Chitosan, a biopolymer, can be used as a template for synthesizing WO3 nanoparticles with enhanced electrochemical properties. []
Q9: How can the morphology of this compound be controlled during synthesis?
A9: The morphology of WO3 can be controlled by adjusting various synthesis parameters, such as:
- Temperature: Higher temperatures generally promote crystal growth and can lead to larger particle sizes. []
- Precursor concentration: Varying the concentration of precursors can influence the nucleation and growth rates of WO3 crystals, affecting their size and shape. [, ]
- pH: Adjusting the pH of the reaction solution can affect the hydrolysis and condensation rates of precursors, influencing particle size and morphology. []
- Use of templates: Utilizing templates like anodic aluminum oxide (AAO) or biopolymers like chitosan can direct the growth of WO3 into specific morphologies, such as nanotubes or nanoparticles. [, ]
- Post-synthesis treatments: Heat treatments like calcination can induce phase transformations and alter the morphology of WO3. [, , ]
Q10: How do lithium ions influence the electrochromic properties of WO3 thin films?
A11: Lithium intercalation into WO3 thin films induces a two-step reduction process, first from W(6+) to W(5+) and then from W(5+) to W(4+). The blue coloration observed in electrochromic WO3 is primarily attributed to the presence of W(4+), with the first intercalation step showing significantly higher coloration efficiency. []
Q11: How does the structure of WO3 thin films affect their electrochromic behavior under dry lithiation?
A12: Studies on nanocrystalline, polycrystalline, and amorphous WO3 films reveal that their electrochromic properties differ significantly under dry lithiation. Nanocrystalline films demonstrate higher overall coloration efficiency and selective optical modulation, particularly in the infrared region, making them suitable for smart window applications. In contrast, amorphous and highly lithiated polycrystalline films exhibit efficient coloration in the visible spectrum, making them suitable for large-area display devices. []
Q12: What are some promising applications of this compound nanostructures?
A12: this compound nanostructures have shown great potential in various applications, including:
- Photocatalysis: Degrading organic pollutants in water and air purification using sunlight. [, , ]
- Electrochromic devices: Smart windows, displays, and other applications requiring controllable light transmission. [, , , ]
- Gas sensors: Detecting various gases, including hydrogen sulfide and nitrogen dioxide. [, ]
- Solar energy conversion: Photoelectrochemical water splitting for hydrogen production. [, ]
- Energy storage: Supercapacitors and other energy storage devices. []
Disclaimer and Information on In-Vitro Research Products
Please be aware that all articles and product information presented on BenchChem are intended solely for informational purposes. The products available for purchase on BenchChem are specifically designed for in-vitro studies, which are conducted outside of living organisms. In-vitro studies, derived from the Latin term "in glass," involve experiments performed in controlled laboratory settings using cells or tissues. It is important to note that these products are not categorized as medicines or drugs, and they have not received approval from the FDA for the prevention, treatment, or cure of any medical condition, ailment, or disease. We must emphasize that any form of bodily introduction of these products into humans or animals is strictly prohibited by law. It is essential to adhere to these guidelines to ensure compliance with legal and ethical standards in research and experimentation.