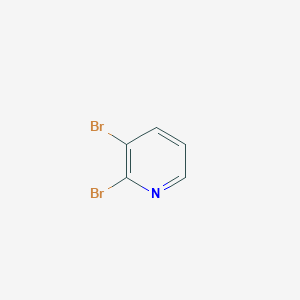
2,3-Dibromopyridine
Overview
Description
2,3-Dibromopyridine is an organic compound with the molecular formula C5H3Br2N. It is a derivative of pyridine, where two bromine atoms are substituted at the 2nd and 3rd positions of the pyridine ring. This compound appears as a white to light yellow crystalline powder and is known for its utility in various chemical syntheses and industrial applications .
Mechanism of Action
Target of Action
As a halogenated pyridine derivative, it may interact with various biological targets, including enzymes and receptors, depending on the specific context of its use .
Mode of Action
Brominated compounds are often used in medicinal chemistry due to their ability to form stable carbon-halogen bonds, which can enhance binding affinity to biological targets .
Biochemical Pathways
Halogenated pyridines are often used as building blocks in the synthesis of complex organic molecules, suggesting that they may influence a variety of biochemical processes .
Pharmacokinetics
The physicochemical properties of the compound, such as its molecular weight (23689 g/mol) and its form (powder), suggest that it may have reasonable bioavailability .
Result of Action
Given its potential to interact with various biological targets, it may have diverse effects at the molecular and cellular levels .
Action Environment
The action, efficacy, and stability of 2,3-Dibromopyridine can be influenced by various environmental factors. These may include the pH and temperature of the environment, the presence of other compounds, and specific conditions within the biological system where it is applied .
Preparation Methods
Synthetic Routes and Reaction Conditions: 2,3-Dibromopyridine can be synthesized through several methods. One common approach involves the bromination of pyridine using bromine or a bromine source in the presence of a catalyst. The reaction typically occurs under controlled conditions to ensure selective bromination at the desired positions .
Industrial Production Methods: In industrial settings, the production of this compound often involves large-scale bromination processes. These processes are optimized for high yield and purity, utilizing advanced techniques such as continuous flow reactors and automated control systems to maintain consistent reaction conditions .
Chemical Reactions Analysis
Types of Reactions: 2,3-Dibromopyridine undergoes various chemical reactions, including:
Substitution Reactions: It can participate in nucleophilic substitution reactions where the bromine atoms are replaced by other nucleophiles.
Coupling Reactions: It is commonly used in Suzuki-Miyaura coupling reactions to form carbon-carbon bonds with boronic acids.
Reduction Reactions: The bromine atoms can be reduced to form pyridine derivatives with different functional groups.
Common Reagents and Conditions:
Nucleophilic Substitution: Reagents such as sodium amide or organolithium compounds are used under anhydrous conditions.
Suzuki-Miyaura Coupling: Palladium catalysts and boronic acids are employed in the presence of a base like potassium carbonate.
Reduction: Reducing agents like lithium aluminum hydride or catalytic hydrogenation are used.
Major Products:
Substitution Reactions: Products include various substituted pyridines.
Coupling Reactions: Products are often biaryl compounds.
Reduction Reactions: Products include partially or fully reduced pyridine derivatives.
Scientific Research Applications
2,3-Dibromopyridine has a wide range of applications in scientific research:
Comparison with Similar Compounds
- 2,4-Dibromopyridine
- 2,5-Dibromopyridine
- 2,6-Dibromopyridine
- 3,5-Dibromopyridine
Comparison: 2,3-Dibromopyridine is unique due to the specific positioning of the bromine atoms, which influences its reactivity and the types of reactions it can undergo. For example, 2,4-Dibromopyridine and 2,6-Dibromopyridine have different substitution patterns, leading to variations in their chemical behavior and applications .
Biological Activity
2,3-Dibromopyridine is a halogenated pyridine derivative known for its unique substitution pattern, which influences both its chemical reactivity and potential biological activities. This compound has garnered attention in medicinal chemistry due to its diverse biological effects, including antimicrobial, antiviral, and cytotoxic properties.
This compound (CHBrN) features two bromine atoms attached to the pyridine ring, which enhances its electrophilic character and reactivity in various chemical reactions. The presence of halogens often modifies the compound's interaction with biological targets.
Antimicrobial Activity
Research indicates that this compound exhibits significant antimicrobial properties. In a study evaluating various pyridine derivatives, this compound demonstrated notable activity against several bacterial strains. The Minimum Inhibitory Concentration (MIC) values were recorded as follows:
Bacterial Strain | MIC (µg/mL) |
---|---|
Staphylococcus aureus | 32 |
Escherichia coli | 64 |
Pseudomonas aeruginosa | 128 |
These findings suggest that the compound could serve as a lead structure for developing new antimicrobial agents .
Antiviral Activity
The antiviral potential of this compound has also been explored. A study focused on its efficacy against viral infections showed that it could inhibit viral replication in vitro. The compound was tested against the influenza virus, yielding an IC50 value of approximately 15 µM. This suggests a promising avenue for further research into its use as an antiviral agent .
Cytotoxic Effects
Cytotoxicity studies reveal that this compound can induce cell death in various cancer cell lines. In particular, it has shown effectiveness against human cancer cell lines such as HeLa and MCF-7. The IC50 values for these lines were reported as:
Cell Line | IC50 (µM) |
---|---|
HeLa | 10 |
MCF-7 | 12 |
These results highlight the compound's potential as a candidate for anticancer drug development .
The biological activity of this compound is attributed to its ability to interact with biological macromolecules. It is believed to inhibit key enzymes involved in cellular processes. For instance, studies suggest that it may act as an inhibitor of protein kinases and other critical enzymes involved in signal transduction pathways .
Case Studies
- Antimicrobial Efficacy : A case study evaluated the effectiveness of this compound against multi-drug resistant strains of bacteria. Results indicated that the compound could restore sensitivity to antibiotics when used in combination therapies.
- Cytotoxicity in Cancer Research : Another study investigated the effects of this compound on tumor growth in xenograft models. The results showed a significant reduction in tumor size compared to control groups when treated with the compound .
Properties
IUPAC Name |
2,3-dibromopyridine | |
---|---|---|
Source | PubChem | |
URL | https://pubchem.ncbi.nlm.nih.gov | |
Description | Data deposited in or computed by PubChem | |
InChI |
InChI=1S/C5H3Br2N/c6-4-2-1-3-8-5(4)7/h1-3H | |
Source | PubChem | |
URL | https://pubchem.ncbi.nlm.nih.gov | |
Description | Data deposited in or computed by PubChem | |
InChI Key |
SLMHHOVQRSSRCV-UHFFFAOYSA-N | |
Source | PubChem | |
URL | https://pubchem.ncbi.nlm.nih.gov | |
Description | Data deposited in or computed by PubChem | |
Canonical SMILES |
C1=CC(=C(N=C1)Br)Br | |
Source | PubChem | |
URL | https://pubchem.ncbi.nlm.nih.gov | |
Description | Data deposited in or computed by PubChem | |
Molecular Formula |
C5H3Br2N | |
Source | PubChem | |
URL | https://pubchem.ncbi.nlm.nih.gov | |
Description | Data deposited in or computed by PubChem | |
DSSTOX Substance ID |
DTXSID40355743 | |
Record name | 2,3-Dibromopyridine | |
Source | EPA DSSTox | |
URL | https://comptox.epa.gov/dashboard/DTXSID40355743 | |
Description | DSSTox provides a high quality public chemistry resource for supporting improved predictive toxicology. | |
Molecular Weight |
236.89 g/mol | |
Source | PubChem | |
URL | https://pubchem.ncbi.nlm.nih.gov | |
Description | Data deposited in or computed by PubChem | |
CAS No. |
13534-89-9 | |
Record name | 2,3-Dibromopyridine | |
Source | EPA DSSTox | |
URL | https://comptox.epa.gov/dashboard/DTXSID40355743 | |
Description | DSSTox provides a high quality public chemistry resource for supporting improved predictive toxicology. | |
Record name | 2,3-Dibromopyridine | |
Source | European Chemicals Agency (ECHA) | |
URL | https://echa.europa.eu/information-on-chemicals | |
Description | The European Chemicals Agency (ECHA) is an agency of the European Union which is the driving force among regulatory authorities in implementing the EU's groundbreaking chemicals legislation for the benefit of human health and the environment as well as for innovation and competitiveness. | |
Explanation | Use of the information, documents and data from the ECHA website is subject to the terms and conditions of this Legal Notice, and subject to other binding limitations provided for under applicable law, the information, documents and data made available on the ECHA website may be reproduced, distributed and/or used, totally or in part, for non-commercial purposes provided that ECHA is acknowledged as the source: "Source: European Chemicals Agency, http://echa.europa.eu/". Such acknowledgement must be included in each copy of the material. ECHA permits and encourages organisations and individuals to create links to the ECHA website under the following cumulative conditions: Links can only be made to webpages that provide a link to the Legal Notice page. | |
Retrosynthesis Analysis
AI-Powered Synthesis Planning: Our tool employs the Template_relevance Pistachio, Template_relevance Bkms_metabolic, Template_relevance Pistachio_ringbreaker, Template_relevance Reaxys, Template_relevance Reaxys_biocatalysis model, leveraging a vast database of chemical reactions to predict feasible synthetic routes.
One-Step Synthesis Focus: Specifically designed for one-step synthesis, it provides concise and direct routes for your target compounds, streamlining the synthesis process.
Accurate Predictions: Utilizing the extensive PISTACHIO, BKMS_METABOLIC, PISTACHIO_RINGBREAKER, REAXYS, REAXYS_BIOCATALYSIS database, our tool offers high-accuracy predictions, reflecting the latest in chemical research and data.
Strategy Settings
Precursor scoring | Relevance Heuristic |
---|---|
Min. plausibility | 0.01 |
Model | Template_relevance |
Template Set | Pistachio/Bkms_metabolic/Pistachio_ringbreaker/Reaxys/Reaxys_biocatalysis |
Top-N result to add to graph | 6 |
Feasible Synthetic Routes
Q1: What makes 2,3-Dibromopyridine particularly useful in organic synthesis?
A1: this compound serves as a valuable building block due to its reactivity in site-selective reactions. The presence of two bromine atoms allows for sequential functionalization, enabling the creation of complex molecules. For instance, it is utilized in synthesizing α- and δ-carbolines, heterocyclic compounds with diverse biological activities [, ].
Q2: How does the nitrogen atom in this compound influence its reactivity?
A2: The nitrogen atom plays a crucial role in directing reactivity. Studies using Density Functional Theory (DFT) calculations have shown that the nitrogen atom activates the carbon atoms at positions 2 and 3, making them more susceptible to oxidative addition reactions with palladium catalysts []. This activation is key for selective functionalization of the pyridine ring.
Q3: Are there any notable examples of regioselective reactions using this compound?
A3: Yes, researchers have successfully demonstrated site-selective Suzuki-Miyaura reactions using this compound. In these reactions, the less sterically hindered bromine atom at position 3 reacts preferentially with arylboronic acids []. This selectivity enables the controlled synthesis of specific isomers, which is crucial for drug discovery and materials science.
Q4: Has the bromine-lithium exchange reaction been explored with this compound?
A4: Yes, researchers have achieved bromine-lithium exchange at the 2-position of this compound under non-cryogenic conditions using TMSCH(2)Li-LiDMAE [, ]. This advancement allows for further functionalization at a relatively accessible temperature, expanding the synthetic utility of this compound.
Q5: Beyond solution-phase chemistry, has this compound been studied on surfaces?
A5: Indeed, researchers have investigated the adsorption and thermal reactions of this compound on a copper (100) surface under ultra-high vacuum conditions []. This surface science study revealed stepwise C-Br bond dissociation, ultimately leading to the formation of a stable pyridine species on the copper surface.
Q6: Are there any known crystal structures involving this compound?
A6: Yes, the crystal structure of 3-Bromopyridine-2-carbonitrile, synthesized from this compound, has been reported []. The crystal packing reveals short intermolecular Br...N contacts and π–π stacking interactions, providing insights into the intermolecular forces involving this compound.
Q7: Has this compound been used in the synthesis of molecules with interesting photophysical properties?
A7: Yes, researchers have synthesized pyrene-based double aza- and diaza[4]helicenes incorporating this compound as a starting material []. These helicenes exhibit pH-sensitive fluorescence, highlighting the potential of this compound as a building block for creating materials with tunable optical properties.
Disclaimer and Information on In-Vitro Research Products
Please be aware that all articles and product information presented on BenchChem are intended solely for informational purposes. The products available for purchase on BenchChem are specifically designed for in-vitro studies, which are conducted outside of living organisms. In-vitro studies, derived from the Latin term "in glass," involve experiments performed in controlled laboratory settings using cells or tissues. It is important to note that these products are not categorized as medicines or drugs, and they have not received approval from the FDA for the prevention, treatment, or cure of any medical condition, ailment, or disease. We must emphasize that any form of bodily introduction of these products into humans or animals is strictly prohibited by law. It is essential to adhere to these guidelines to ensure compliance with legal and ethical standards in research and experimentation.