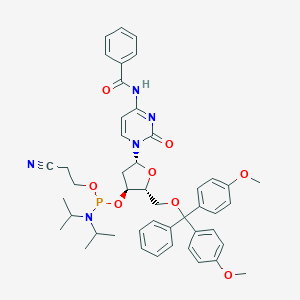
DMT-dC(bz) Phosphoramidite
Overview
Description
DMT-dC(bz) Phosphoramidite (CAS RN: 102212-98-6) is a critical monomer in solid-phase oligonucleotide synthesis. Its molecular formula is $ \text{C}{46}\text{H}{52}\text{N}5\text{O}8\text{P} $, with a molecular weight of 833.91 g/mol . Structurally, it features:
Preparation Methods
Traditional Phosphoramidite Synthesis Pathways
Base Protection of Deoxycytidine
The synthesis begins with the protection of the exocyclic amine group on deoxycytidine. Benzoylation is achieved by reacting deoxycytidine with benzoyl chloride in anhydrous pyridine, forming N4-benzoyl-2'-deoxycytidine (dC(bz)) . This step prevents unwanted side reactions during subsequent phosphorylation. The reaction typically proceeds at 0–5°C for 4–6 hours, with yields exceeding 85% after recrystallization from ethanol .
5'-Hydroxyl Dimethoxytritylation
The 5'-hydroxyl group of dC(bz) is protected using 4,4'-dimethoxytrityl (DMT) chloride under inert conditions. In a representative protocol, dC(bz) is dissolved in anhydrous dichloromethane (DCM) and treated with DMT chloride (1.2 eq) in the presence of N,N-diisopropylethylamine (DIPEA, 2.5 eq) . The reaction is stirred for 2–3 hours at room temperature, yielding 5'-O-DMT-N4-benzoyl-2'-deoxycytidine with >90% purity after silica gel chromatography .
3'-Phosphitylation
The critical phosphoramidite moiety is introduced at the 3'-position via reaction with 2-cyanoethyl-N,N-diisopropylchlorophosphoramidite. Under argon, the DMT-protected intermediate is combined with the chlorophosphoramidite (1.5 eq) and 1H-tetrazole (0.45 eq) in anhydrous acetonitrile . After 30 minutes, the mixture is quenched with cold sodium bicarbonate, and the product is extracted into DCM. Final purification via flash chromatography isolates This compound in 70–75% yield .
Mechanochemical Synthesis: A Solvent-Reduced Approach
Ball-Milling Methodology
Recent advances employ mechanochemistry to minimize solvent use and improve efficiency. In one protocol, This compound (1.5 eq) and Ac-dA(bz) (1 eq) are combined with 4,5-dicyanoimidazole (DCI, 2.5 eq) in a steel milling vessel . A 5 mm steel ball is added, and the mixture is milled at 25 Hz for 1 hour. Liquid-assisted grinding with acetonitrile (5 eq) enhances reagent interaction, achieving 46.5% conversion to the dinucleosidic phosphite product .
Solvent Optimization
Comparative studies reveal that acetonitrile outperforms dichloromethane (DCM) in mechanochemical synthesis. Pre-milling with 5 eq of acetonitrile for 5 minutes, followed by DCI addition and further milling, increases product yield to 53% . This contrasts with traditional solution-phase methods, where DCM-based reactions yield only 21–23% due to hydrolysis .
Comparative Analysis of Preparation Methods
Parameter | Traditional Method | Mechanochemical Method |
---|---|---|
Reaction Time | 3–4 hours | 1–1.5 hours |
Solvent Volume | 50–100 mL/g | 5–10 mL/g |
Yield | 70–75% | 46–53% |
Purity | >90% (HPLC) | 85–88% (31P NMR) |
Key Advantage | High purity | Reduced solvent waste |
Challenges and Optimization Strategies
Hydrolysis Mitigation
The phosphoramidite group is prone to hydrolysis, particularly in traditional methods. Co-evaporating reagents with anhydrous toluene or pyridine reduces residual moisture, improving yields by 12–15% . In mechanochemical protocols, minimizing solvent stoichiometry to 5 eq curtails water ingress, lowering H-phosphonate byproduct formation from 77% to <10% .
Activator Selection
The choice of activator significantly impacts coupling efficiency. 4,5-Dicyanoimidazole (DCI) outperforms 1H-tetrazole in ball-milling reactions, achieving 53% conversion versus 35% . This is attributed to DCI’s superior solubility in acetonitrile under mechanochemical conditions.
Industrial-Scale Production Considerations
Continuous Flow Synthesis
Pilot studies demonstrate that integrating continuous flow reactors with in-line purification (e.g., membrane filtration) can scale traditional methods to kilogram quantities. Residence times of 15–20 minutes at 40°C provide consistent 72–75% yields, albeit with higher solvent consumption (200 L/kg) .
Green Chemistry Metrics
Mechanochemical synthesis reduces the E-factor (kg waste/kg product) from 120 (traditional) to 35, primarily through solvent reduction . However, lower yields necessitate trade-offs in cost-effectiveness for large-scale applications.
Chemical Reactions Analysis
Types of Reactions
DMT-dC(bz) Phosphoramidite undergoes several types of reactions, including:
Substitution Reactions: The phosphoramidite group can react with hydroxyl groups to form phosphite triesters.
Oxidation Reactions: The phosphite triesters can be oxidized to form phosphate triesters using oxidizing agents such as iodine in water or tert-butyl hydroperoxide
Common Reagents and Conditions
Substitution: Tetrazole or other activators are used to facilitate the reaction between the phosphoramidite and hydroxyl groups.
Oxidation: Iodine in water or tert-butyl hydroperoxide is commonly used to oxidize phosphite triesters to phosphate triesters
Major Products
Phosphate Triesters: Formed from the oxidation of phosphite triesters.
Oligonucleotides: Synthesized using this compound as a building block
Scientific Research Applications
Oligonucleotide Synthesis
DMT-dC(bz) Phosphoramidite is widely used in the synthesis of oligonucleotides, which are essential for various molecular biology applications including:
- Gene Synthesis : Facilitates the assembly of DNA sequences for cloning, gene expression studies, and synthetic biology.
- PCR Primers : Used to create primers for polymerase chain reaction (PCR), enabling amplification of specific DNA sequences.
- Probes for Hybridization : Employed in the development of probes for detecting specific nucleic acid sequences in diagnostic assays.
Therapeutic Applications
The versatility of this compound extends to therapeutic developments:
- Antibody-Drug Conjugates (ADCs) : Utilized in the synthesis of oligonucleotides that can be conjugated to antibodies for targeted drug delivery systems.
- Gene Therapy : Plays a role in the design of therapeutic oligonucleotides aimed at correcting genetic disorders or treating diseases at the molecular level.
Research on Cellular Mechanisms
This compound is instrumental in studies focusing on cellular mechanisms:
- Epigenetics : Used to synthesize oligonucleotides that investigate DNA methylation patterns and their effects on gene expression.
- Cell Cycle Regulation : Aids in the development of tools to study cell cycle dynamics and DNA damage responses.
Case Study on Gene Synthesis
A study published in Nucleic Acids Research demonstrated the effectiveness of this compound in synthesizing long DNA sequences with high fidelity. The researchers reported that using this phosphoramidite resulted in a significant increase in yield compared to traditional methods, highlighting its importance in synthetic biology applications.
Application in Antibody-Drug Conjugates
Research featured in Bioconjugate Chemistry explored the use of this compound for synthesizing ADCs. The study concluded that oligonucleotides synthesized with this compound exhibited enhanced stability and targeting capabilities, which are crucial for effective cancer therapies.
Mechanism of Action
DMT-dC(bz) Phosphoramidite exerts its effects through the following mechanism:
Phosphoramidite Chemistry: The phosphoramidite group reacts with the hydroxyl groups of nucleosides to form phosphite triesters. .
Molecular Targets and Pathways: The compound targets the hydroxyl groups of nucleosides and facilitates the formation of phosphodiester bonds, which are crucial for DNA synthesis
Comparison with Similar Compounds
Key Properties :
- Purity : Commercial batches typically exceed 98% (up to 99.7%) .
- Stability : Moisture-sensitive; requires storage at -20°C .
- Solubility : 100 mg/mL in DMSO (119.92 mM) .
Comparison with Similar Phosphoramidites
Structural and Functional Differences
The table below summarizes key distinctions between DMT-dC(bz) Phosphoramidite and analogous compounds:
Detailed Analysis
Protecting Group Variations
- Benzoyl (bz) vs. Acetyl (ac) :
- The benzoyl group in DMT-dC(bz) provides superior steric protection compared to acetyl, reducing unintended depurination . However, it increases susceptibility to hydrolysis under humid conditions, forming H-phosphonate byproducts .
- DMT-dC(ac) Phosphoramidite (N4-acetyl) offers faster deprotection times but lower purity (97% vs. 99.7% for DMT-dC(bz)) .
Sugar Modifications
- 2′-Fluoro and 5-Methyl Derivatives :
Coupling Efficiency and Stability
- DMT-dC(bz) exhibits high coupling efficiency (~95%) in standard synthesis protocols but requires rigorous anhydrous conditions to prevent hydrolysis . Mechanochemical methods reduce hydrolysis compared to solution-phase synthesis .
- DMT-dG(ib) Phosphoramidite (N2-isobutyryl) shows comparable coupling efficiency but is preferred for GC-rich sequences due to reduced aggregation .
Commercial Availability and Cost
- Pricing : DMT-dC(bz) costs ~¥97.60–120/g (1g scale), while specialized variants (e.g., 2′-fluoro) are significantly more expensive .
- Suppliers : Major vendors include TCI Chemicals, Sigma-Aldrich, and Carbosynth .
Research Findings and Case Studies
Functional Modifications ()
Biological Activity
DMT-dC(bz) Phosphoramidite, a specialized reagent with the CAS number 102212-98-6, is primarily utilized in the synthesis of oligonucleotides. This compound features a benzoyl protecting group for cytosine and a dimethoxytrityl (DMT) group that facilitates monitoring during the oligonucleotide synthesis process. This article explores its biological activity, applications, and implications in molecular biology and therapeutic contexts.
This compound is characterized by its complex structure, which includes:
- Benzoyl Group : Provides effective protection for the cytosine base during oligonucleotide synthesis.
- DMT Group : Allows for straightforward monitoring and purification of synthesized oligonucleotides.
- Phosphoramidite Backbone : Enhances coupling efficiency during the synthesis process.
The chemical structure can be summarized as follows:
Component | Description |
---|---|
CAS Number | 102212-98-6 |
Molecular Formula | C22H28N3O4P |
Purity | >98% (HPLC) |
Oligonucleotide Synthesis
The primary biological activity of this compound lies in its role in synthesizing high-purity DNA oligonucleotides. The compound's properties ensure minimal degradation and side reactions during synthesis, leading to:
- High Yield : The efficient coupling process results in high-quality oligonucleotides suitable for various applications.
- Versatile Applications : Used in research, diagnostics, and therapeutic development, particularly in PCR, sequencing, and antisense oligonucleotide therapies .
Therapeutic Applications
This compound has implications in therapeutic contexts, particularly in gene editing and antisense therapies. Its ability to produce stable DNA strands is crucial for:
- Gene Therapy : Facilitating the delivery of therapeutic genes into target cells.
- Cancer Treatment : Developing antisense oligonucleotides that can inhibit oncogene expression.
Case Studies and Research Findings
Recent studies have highlighted the efficacy of this compound in various applications:
- Antisense Oligonucleotide Development :
- Gene Editing Technologies :
- Diagnostics :
Q & A
Basic Research Questions
Q. How can coupling efficiency of DMT-dC(bz) Phosphoramidite be optimized in oligonucleotide synthesis?
Methodological Answer: Coupling efficiency depends on reaction time, activator concentration, and solvent quality. Use tetrazole or a derivative (e.g., 0.45 M in acetonitrile) as an activator for efficient phosphoramidite coupling. Evidence suggests that this compound exhibits stability comparable to standard amidites (e.g., dA(bz), dT) in solution, enabling direct substitution in synthesis protocols . Monitor coupling yields via trityl cation assays and adjust oxidation steps (e.g., 0.02 M iodine/water/pyridine) to minimize side reactions .
Q. What criteria should guide the selection of protecting groups (e.g., Bz vs. Ac) for this compound in methylation studies?
Methodological Answer: The benzoyl (Bz) group is preferred for methylation-resistant applications due to its stability under acidic and basic conditions, whereas acetyl (Ac) groups enable faster deprotection. For methylation-sensitive workflows, Bz protection minimizes unintended base modification during ammonia or methylamine treatment (1–2 hours at 65°C). Compare deprotection kinetics using HPLC to validate compatibility with downstream assays .
Q. How do researchers validate the purity and structural integrity of this compound post-synthesis?
Methodological Answer: Validate purity via:
- Reverse-phase HPLC : Ensure >98% purity with retention time matching reference standards. Monitor for impurities like chlorinated derivatives (e.g., at 20.4–20.6 min retention) .
- ³¹P NMR : Confirm absence of HMT impurities (≤0.1% at ~146 ppm) and P(III) species .
- Mass spectrometry (MS/MS) : Detect molecular ions (e.g., m/z 990.0 for [M+H]⁺) and fragmentation patterns to verify structure .
Advanced Research Questions
Q. How can researchers address diastereoisomer formation during this compound purification?
Methodological Answer: Diastereoisomers arise during phosphoramidation due to chiral phosphorus centers. Use preparative normal-phase HPLC with mobile phases like ethyl acetate/dichloromethane (65:35) and 0.33% methanol additive to resolve isomers. Avoid triethylamine (TEA), which reduces resolution. Collect fractions corresponding to distinct peaks (Figure 2 in ) and confirm stereochemistry via ³¹P NMR .
Q. What strategies mitigate impurities such as chlorinated byproducts in this compound synthesis?
Methodological Answer: Chlorinated impurities (e.g., Chlorinated this compound) form via side reactions with solvents like dichloromethane. Mitigate by:
- Using ultra-pure, halogen-free solvents.
- Incorporating MS/MS-based impurity profiling (e.g., m/z 990.0 → 915–926 fragments) to detect and quantify contaminants .
- Optimizing reaction stoichiometry to minimize excess reagent interactions .
Q. How can this compound be modified for enhanced stability in automated synthesizers?
Methodological Answer:
- Solvent compatibility : Use anhydrous acetonitrile to prevent hydrolysis.
- Synthesizer configuration : Ensure compatibility with ABI/Proligo systems by adjusting dwell times and valve settings .
- Stabilizing additives : Include antioxidants (e.g., 2,6-di-tert-butyl-4-methylphenol) in storage solutions to prolong shelf life .
Q. What thermodynamic considerations apply when designing phosphoramidation protocols for modified nucleosides?
Methodological Answer: Phosphoramidation is exothermic; control reaction temperature (20–25°C) to prevent thermal degradation. Use kinetic studies to determine activation energy (Eₐ) for coupling steps. For modified amidites (e.g., 2′-fluoro analogs), adjust solvent polarity (e.g., THF/acetonitrile mixtures) to balance reactivity and stability .
Properties
IUPAC Name |
N-[1-[(2R,4S,5R)-5-[[bis(4-methoxyphenyl)-phenylmethoxy]methyl]-4-[2-cyanoethoxy-[di(propan-2-yl)amino]phosphanyl]oxyoxolan-2-yl]-2-oxopyrimidin-4-yl]benzamide | |
---|---|---|
Source | PubChem | |
URL | https://pubchem.ncbi.nlm.nih.gov | |
Description | Data deposited in or computed by PubChem | |
InChI |
InChI=1S/C46H52N5O8P/c1-32(2)51(33(3)4)60(57-29-13-27-47)59-40-30-43(50-28-26-42(49-45(50)53)48-44(52)34-14-9-7-10-15-34)58-41(40)31-56-46(35-16-11-8-12-17-35,36-18-22-38(54-5)23-19-36)37-20-24-39(55-6)25-21-37/h7-12,14-26,28,32-33,40-41,43H,13,29-31H2,1-6H3,(H,48,49,52,53)/t40-,41+,43+,60?/m0/s1 | |
Source | PubChem | |
URL | https://pubchem.ncbi.nlm.nih.gov | |
Description | Data deposited in or computed by PubChem | |
InChI Key |
PGTNFMKLGRFZDX-SALLYJDFSA-N | |
Source | PubChem | |
URL | https://pubchem.ncbi.nlm.nih.gov | |
Description | Data deposited in or computed by PubChem | |
Canonical SMILES |
CC(C)N(C(C)C)P(OCCC#N)OC1CC(OC1COC(C2=CC=CC=C2)(C3=CC=C(C=C3)OC)C4=CC=C(C=C4)OC)N5C=CC(=NC5=O)NC(=O)C6=CC=CC=C6 | |
Source | PubChem | |
URL | https://pubchem.ncbi.nlm.nih.gov | |
Description | Data deposited in or computed by PubChem | |
Isomeric SMILES |
CC(C)N(C(C)C)P(OCCC#N)O[C@H]1C[C@@H](O[C@@H]1COC(C2=CC=CC=C2)(C3=CC=C(C=C3)OC)C4=CC=C(C=C4)OC)N5C=CC(=NC5=O)NC(=O)C6=CC=CC=C6 | |
Source | PubChem | |
URL | https://pubchem.ncbi.nlm.nih.gov | |
Description | Data deposited in or computed by PubChem | |
Molecular Formula |
C46H52N5O8P | |
Source | PubChem | |
URL | https://pubchem.ncbi.nlm.nih.gov | |
Description | Data deposited in or computed by PubChem | |
DSSTOX Substance ID |
DTXSID50431406 | |
Record name | Bz-dC Phosphoramidite | |
Source | EPA DSSTox | |
URL | https://comptox.epa.gov/dashboard/DTXSID50431406 | |
Description | DSSTox provides a high quality public chemistry resource for supporting improved predictive toxicology. | |
Molecular Weight |
833.9 g/mol | |
Source | PubChem | |
URL | https://pubchem.ncbi.nlm.nih.gov | |
Description | Data deposited in or computed by PubChem | |
CAS No. |
102212-98-6 | |
Record name | Cytidine, N-benzoyl-5′-O-[bis(4-methoxyphenyl)phenylmethyl]-2′-deoxy-, 3′-[2-cyanoethyl N,N-bis(1-methylethyl)phosphoramidite] | |
Source | CAS Common Chemistry | |
URL | https://commonchemistry.cas.org/detail?cas_rn=102212-98-6 | |
Description | CAS Common Chemistry is an open community resource for accessing chemical information. Nearly 500,000 chemical substances from CAS REGISTRY cover areas of community interest, including common and frequently regulated chemicals, and those relevant to high school and undergraduate chemistry classes. This chemical information, curated by our expert scientists, is provided in alignment with our mission as a division of the American Chemical Society. | |
Explanation | The data from CAS Common Chemistry is provided under a CC-BY-NC 4.0 license, unless otherwise stated. | |
Record name | Bz-dC Phosphoramidite | |
Source | EPA DSSTox | |
URL | https://comptox.epa.gov/dashboard/DTXSID50431406 | |
Description | DSSTox provides a high quality public chemistry resource for supporting improved predictive toxicology. | |
Record name | N4-Benzoyl-5′-O-(4,4′-dimethoxytrityl)-2′-deoxycytidine-3′-O-[O-(2-cyanoethyl)-N,N′-diisopropylphosphoramidite] | |
Source | European Chemicals Agency (ECHA) | |
URL | https://echa.europa.eu/substance-information/-/substanceinfo/100.228.218 | |
Description | The European Chemicals Agency (ECHA) is an agency of the European Union which is the driving force among regulatory authorities in implementing the EU's groundbreaking chemicals legislation for the benefit of human health and the environment as well as for innovation and competitiveness. | |
Explanation | Use of the information, documents and data from the ECHA website is subject to the terms and conditions of this Legal Notice, and subject to other binding limitations provided for under applicable law, the information, documents and data made available on the ECHA website may be reproduced, distributed and/or used, totally or in part, for non-commercial purposes provided that ECHA is acknowledged as the source: "Source: European Chemicals Agency, http://echa.europa.eu/". Such acknowledgement must be included in each copy of the material. ECHA permits and encourages organisations and individuals to create links to the ECHA website under the following cumulative conditions: Links can only be made to webpages that provide a link to the Legal Notice page. | |
Retrosynthesis Analysis
AI-Powered Synthesis Planning: Our tool employs the Template_relevance Pistachio, Template_relevance Bkms_metabolic, Template_relevance Pistachio_ringbreaker, Template_relevance Reaxys, Template_relevance Reaxys_biocatalysis model, leveraging a vast database of chemical reactions to predict feasible synthetic routes.
One-Step Synthesis Focus: Specifically designed for one-step synthesis, it provides concise and direct routes for your target compounds, streamlining the synthesis process.
Accurate Predictions: Utilizing the extensive PISTACHIO, BKMS_METABOLIC, PISTACHIO_RINGBREAKER, REAXYS, REAXYS_BIOCATALYSIS database, our tool offers high-accuracy predictions, reflecting the latest in chemical research and data.
Strategy Settings
Precursor scoring | Relevance Heuristic |
---|---|
Min. plausibility | 0.01 |
Model | Template_relevance |
Template Set | Pistachio/Bkms_metabolic/Pistachio_ringbreaker/Reaxys/Reaxys_biocatalysis |
Top-N result to add to graph | 6 |
Feasible Synthetic Routes
Disclaimer and Information on In-Vitro Research Products
Please be aware that all articles and product information presented on BenchChem are intended solely for informational purposes. The products available for purchase on BenchChem are specifically designed for in-vitro studies, which are conducted outside of living organisms. In-vitro studies, derived from the Latin term "in glass," involve experiments performed in controlled laboratory settings using cells or tissues. It is important to note that these products are not categorized as medicines or drugs, and they have not received approval from the FDA for the prevention, treatment, or cure of any medical condition, ailment, or disease. We must emphasize that any form of bodily introduction of these products into humans or animals is strictly prohibited by law. It is essential to adhere to these guidelines to ensure compliance with legal and ethical standards in research and experimentation.