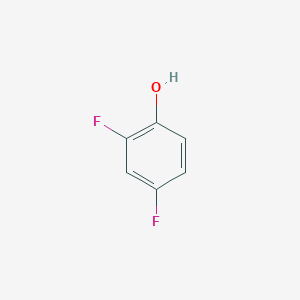
2,4-Difluorophenol
Overview
Description
2,4-Difluorophenol (2,4-DFP) is a phenolic compound with two fluorine atoms attached to the aromatic ring. It is a white crystalline solid that is soluble in organic solvents and has a melting point of 152-154°C. 2,4-DFP is a versatile compound that has a wide variety of applications in scientific research and industry. It is used as a synthetic intermediate in the manufacture of pharmaceuticals, agrochemicals, and other organic compounds. Its unique properties make it an attractive option for a range of scientific and industrial applications.
Scientific Research Applications
Organic Light-Emitting Devices : A study by Li et al. (2012) in "Chemical Physics Letters" found that 2,4-difluorophenyl-functionalized arylamine (4FDT) can enhance the efficiency and luminance of organic light-emitting devices by balancing injected carriers and reducing hole mobility (Li et al., 2012).
Two-Photon Photoexcitation : Shivatare and Tzeng (2014) in "Bulletin of The Korean Chemical Society" discovered that the cis form of 2,4-difluorophenol participates in two-photon photoexcitation and pulsed field ionization processes (Shivatare & Tzeng, 2014).
Environmental Science Implications : Wang et al. (2015) reported in "RSC Advances" that 2,4-dichlorophenol toxicity in aerobic granular sludge systems affects biological nitrogen removal performance, highlighting its environmental impact (Wang et al., 2015).
Drug Development : Qiu et al. (1999) in the "Journal of medicinal chemistry" found that the 2,6-difluorophenol moiety can act as a lipophilic bioisostere of a carboxylic acid, useful in increasing the lipophilicity of drug candidates (Qiu et al., 1999).
Molecular Spectroscopy : Research by Nair et al. (2017) in the "Journal of Molecular Spectroscopy" focused on the supersonic jet-cooled rotational spectrum of this compound, providing insights into its molecular structure (Nair et al., 2017).
Analytical Chemistry : A study by Han et al. (2021) in "Daxue Huaxue" recommended a spectrophotometric method for determining 2,4-dichlorophenol in technical materials, enhancing experimental skills in analytical chemistry (Han et al., 2021).
Mechanism of Action
Target of Action
Similar compounds often interact with enzymes or receptors in the body, altering their function .
Mode of Action
It’s known that many phenolic compounds can interact with biological systems in various ways, such as disrupting membrane function, inhibiting enzyme activity, or acting as antioxidants .
Biochemical Pathways
Phenolic compounds, in general, can influence a variety of biochemical pathways depending on their specific targets .
Pharmacokinetics
Many small, lipophilic molecules like 2,4-difluorophenol are often well-absorbed and can distribute throughout the body .
Result of Action
Phenolic compounds can have a variety of effects at the molecular and cellular level, depending on their specific targets and mode of action .
Action Environment
Environmental factors can influence the action, efficacy, and stability of this compound. Factors such as pH, temperature, and the presence of other substances can affect the compound’s stability and its interactions with biological targets .
Biochemical Analysis
Biochemical Properties
It is known that fluorinated compounds can interact with various enzymes, proteins, and other biomolecules . The nature of these interactions is largely dependent on the specific biochemical context and the other molecules involved .
Molecular Mechanism
It is possible that it exerts its effects at the molecular level through binding interactions with biomolecules, enzyme inhibition or activation, and changes in gene expression .
Dosage Effects in Animal Models
The effects of 2,4-Difluorophenol at different dosages in animal models have not been extensively studied. Therefore, information on threshold effects, as well as toxic or adverse effects at high doses, is currently unavailable .
Transport and Distribution
Information on how this compound is transported and distributed within cells and tissues, including any transporters or binding proteins it interacts with, and any effects on its localization or accumulation, is currently unavailable .
properties
IUPAC Name |
2,4-difluorophenol | |
---|---|---|
Source | PubChem | |
URL | https://pubchem.ncbi.nlm.nih.gov | |
Description | Data deposited in or computed by PubChem | |
InChI |
InChI=1S/C6H4F2O/c7-4-1-2-6(9)5(8)3-4/h1-3,9H | |
Source | PubChem | |
URL | https://pubchem.ncbi.nlm.nih.gov | |
Description | Data deposited in or computed by PubChem | |
InChI Key |
NVWVWEWVLBKPSM-UHFFFAOYSA-N | |
Source | PubChem | |
URL | https://pubchem.ncbi.nlm.nih.gov | |
Description | Data deposited in or computed by PubChem | |
Canonical SMILES |
C1=CC(=C(C=C1F)F)O | |
Source | PubChem | |
URL | https://pubchem.ncbi.nlm.nih.gov | |
Description | Data deposited in or computed by PubChem | |
Molecular Formula |
C6H4F2O | |
Source | PubChem | |
URL | https://pubchem.ncbi.nlm.nih.gov | |
Description | Data deposited in or computed by PubChem | |
DSSTOX Substance ID |
DTXSID50190142 | |
Record name | 2,4-Difluorophenol | |
Source | EPA DSSTox | |
URL | https://comptox.epa.gov/dashboard/DTXSID50190142 | |
Description | DSSTox provides a high quality public chemistry resource for supporting improved predictive toxicology. | |
Molecular Weight |
130.09 g/mol | |
Source | PubChem | |
URL | https://pubchem.ncbi.nlm.nih.gov | |
Description | Data deposited in or computed by PubChem | |
CAS RN |
367-27-1 | |
Record name | 2,4-Difluorophenol | |
Source | CAS Common Chemistry | |
URL | https://commonchemistry.cas.org/detail?cas_rn=367-27-1 | |
Description | CAS Common Chemistry is an open community resource for accessing chemical information. Nearly 500,000 chemical substances from CAS REGISTRY cover areas of community interest, including common and frequently regulated chemicals, and those relevant to high school and undergraduate chemistry classes. This chemical information, curated by our expert scientists, is provided in alignment with our mission as a division of the American Chemical Society. | |
Explanation | The data from CAS Common Chemistry is provided under a CC-BY-NC 4.0 license, unless otherwise stated. | |
Record name | 2,4-Difluorophenol | |
Source | ChemIDplus | |
URL | https://pubchem.ncbi.nlm.nih.gov/substance/?source=chemidplus&sourceid=0000367271 | |
Description | ChemIDplus is a free, web search system that provides access to the structure and nomenclature authority files used for the identification of chemical substances cited in National Library of Medicine (NLM) databases, including the TOXNET system. | |
Record name | 2,4-Difluorophenol | |
Source | EPA DSSTox | |
URL | https://comptox.epa.gov/dashboard/DTXSID50190142 | |
Description | DSSTox provides a high quality public chemistry resource for supporting improved predictive toxicology. | |
Record name | 2,4-difluorophenol | |
Source | European Chemicals Agency (ECHA) | |
URL | https://echa.europa.eu/substance-information/-/substanceinfo/100.006.081 | |
Description | The European Chemicals Agency (ECHA) is an agency of the European Union which is the driving force among regulatory authorities in implementing the EU's groundbreaking chemicals legislation for the benefit of human health and the environment as well as for innovation and competitiveness. | |
Explanation | Use of the information, documents and data from the ECHA website is subject to the terms and conditions of this Legal Notice, and subject to other binding limitations provided for under applicable law, the information, documents and data made available on the ECHA website may be reproduced, distributed and/or used, totally or in part, for non-commercial purposes provided that ECHA is acknowledged as the source: "Source: European Chemicals Agency, http://echa.europa.eu/". Such acknowledgement must be included in each copy of the material. ECHA permits and encourages organisations and individuals to create links to the ECHA website under the following cumulative conditions: Links can only be made to webpages that provide a link to the Legal Notice page. | |
Retrosynthesis Analysis
AI-Powered Synthesis Planning: Our tool employs the Template_relevance Pistachio, Template_relevance Bkms_metabolic, Template_relevance Pistachio_ringbreaker, Template_relevance Reaxys, Template_relevance Reaxys_biocatalysis model, leveraging a vast database of chemical reactions to predict feasible synthetic routes.
One-Step Synthesis Focus: Specifically designed for one-step synthesis, it provides concise and direct routes for your target compounds, streamlining the synthesis process.
Accurate Predictions: Utilizing the extensive PISTACHIO, BKMS_METABOLIC, PISTACHIO_RINGBREAKER, REAXYS, REAXYS_BIOCATALYSIS database, our tool offers high-accuracy predictions, reflecting the latest in chemical research and data.
Strategy Settings
Precursor scoring | Relevance Heuristic |
---|---|
Min. plausibility | 0.01 |
Model | Template_relevance |
Template Set | Pistachio/Bkms_metabolic/Pistachio_ringbreaker/Reaxys/Reaxys_biocatalysis |
Top-N result to add to graph | 6 |
Feasible Synthetic Routes
Disclaimer and Information on In-Vitro Research Products
Please be aware that all articles and product information presented on BenchChem are intended solely for informational purposes. The products available for purchase on BenchChem are specifically designed for in-vitro studies, which are conducted outside of living organisms. In-vitro studies, derived from the Latin term "in glass," involve experiments performed in controlled laboratory settings using cells or tissues. It is important to note that these products are not categorized as medicines or drugs, and they have not received approval from the FDA for the prevention, treatment, or cure of any medical condition, ailment, or disease. We must emphasize that any form of bodily introduction of these products into humans or animals is strictly prohibited by law. It is essential to adhere to these guidelines to ensure compliance with legal and ethical standards in research and experimentation.