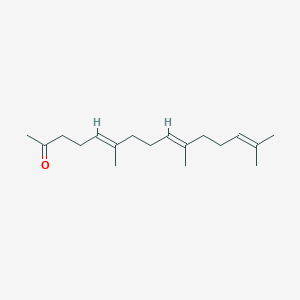
Farnesylacetone
Overview
Description
Synthesis Analysis
Farnesylacetone synthesis involves sophisticated chemical processes that yield its unique structure. Notably, the synthesis of farnesol isomers, a closely related compound, from nerylacetone demonstrates the versatility of synthetic approaches through modified Wittig procedures and Horner-Wadsworth-Emmons condensation, providing a foundation for this compound synthesis (Yu, Kleckley, & Wiemer, 2005).
Molecular Structure Analysis
The molecular structure of this compound is critical to its biological function and chemical behavior. Its structure has been elucidated through various analytical techniques, showcasing its sesquiterpenic nature which contributes to its biological activities and interactions within biological systems.
Chemical Reactions and Properties
This compound participates in several chemical reactions, indicating its reactivity and functional role in biological processes. For example, it has been shown to inhibit vitellogenesis in crustaceans by affecting macromolecular synthesis in the gonads, demonstrating its gonad-specific action that varies with species (Berreur-Bonnenfant & Lawrence, 1984).
Physical Properties Analysis
The physical properties of this compound, such as solubility, boiling point, and melting point, are essential for understanding its behavior in different environments, including biological systems. These properties influence its method of isolation, purification, and application in research and development.
Chemical Properties Analysis
The chemical properties of this compound, including its reactivity with other molecules and its stability under various conditions, are crucial for its biological and chemical applications. Its role as a sesquiterpenic hormone in crustacea, for instance, highlights its significant impact on electron transport in isolated rat liver mitochondria, affecting cellular respiration and energy production (Dupont et al., 1989).
Scientific Research Applications
Mitochondrial Electron Transport Inhibition : Farnesylacetone inhibits electron transport in isolated rat liver mitochondria, potentially interacting with the respiratory chain at the iron-sulfur centers (Dupont et al., 1989).
Impact on Lipid Metabolism : It causes an increase in neutral lipidic droplets in the cytoplasm and alters lipid metabolism in transformed rat fibroblasts, also temporarily increasing membrane fluidity (Rodes et al., 1995).
Biological Activity in Crustacean Ovaries : this compound shows biological activity by inhibiting the incorporation of 3H-leucine in Crustacean ovaries subcultures (Férézou et al., 1977).
Protein Synthesis in Ovaries : It activates protein synthesis in the ovary of the crab Carcinus maenas during summer (Andrieux et al., 1989).
Transcription Modification in Crustacean Gonads : this compound modifies transcription and only indirectly affects protein synthesis in the gonads of crustaceans (Berreur-Bonnenfant & Lawrence, 1984).
Inhibition of Biological Methylations : T,t-farnesylacetone inhibits the methylation of E. coli B tRNA and Calf thymus histones in vitro (Tekitek et al., 1977).
Protein Farnesylation in Embryogenesis and Cancer : Protein farnesylation, a process involving this compound, is essential for early embryogenesis and critical for tumor progression, but not initiation (Sebti, 2005).
Vasodilatation Effects : Farnesylacetones from Sargassum siliquastrum show vasodilatation effects, greater selectivity toward L-type Ca(2+) channels, and potent, long-lasting antihypertensive activity in rats (Shin et al., 2013).
Effect on Cerebral Blood Flow : They may accelerate cerebral blood flow through dilation of the basilar artery (Park et al., 2008).
Marker for Cystophora Species : 9,10-farnesylacetone epoxide may be an intra-specific marker for certain Cystophora species populations (Laird et al., 2010).
Mechanism of Action
Target of Action
Farnesylacetone has been found to exhibit inhibitory activities against acetylcholinesterase and butyrylcholinesterase . These enzymes play crucial roles in the nervous system, particularly in the breakdown of acetylcholine, a neurotransmitter essential for memory and cognition. Inhibition of these enzymes can lead to an increase in acetylcholine levels, which may have therapeutic implications for conditions like Alzheimer’s disease .
Mode of Action
This results in increased levels of acetylcholine in the synaptic cleft, enhancing cholinergic transmission .
Biochemical Pathways
This compound is part of the terpene family, which are key components of the isoprenoid pathway . This pathway is responsible for the production of a wide range of compounds, including hormones, pigments, and volatiles. The exact biochemical pathways affected by this compound and their downstream effects are still under investigation.
Result of Action
The primary result of this compound’s action is the inhibition of acetylcholinesterase and butyrylcholinesterase, leading to increased acetylcholine levels . This can enhance cholinergic transmission, which may have beneficial effects on cognitive function.
Safety and Hazards
Future Directions
Farnesylacetone and its derivatives are important starting compounds for natural and artificial organic synthesis . It is also present in many essential oils such as citronella, neroli, cyclamen, lemon grass, tuberose, rose, musk, balsam, and tolu . It is used in perfumery to emphasize the odors of sweet, floral perfumes .
properties
IUPAC Name |
(5E,9E)-6,10,14-trimethylpentadeca-5,9,13-trien-2-one | |
---|---|---|
Source | PubChem | |
URL | https://pubchem.ncbi.nlm.nih.gov | |
Description | Data deposited in or computed by PubChem | |
InChI |
InChI=1S/C18H30O/c1-15(2)9-6-10-16(3)11-7-12-17(4)13-8-14-18(5)19/h9,11,13H,6-8,10,12,14H2,1-5H3/b16-11+,17-13+ | |
Source | PubChem | |
URL | https://pubchem.ncbi.nlm.nih.gov | |
Description | Data deposited in or computed by PubChem | |
InChI Key |
LTUMRKDLVGQMJU-IUBLYSDUSA-N | |
Source | PubChem | |
URL | https://pubchem.ncbi.nlm.nih.gov | |
Description | Data deposited in or computed by PubChem | |
Canonical SMILES |
CC(=CCCC(=CCCC(=CCCC(=O)C)C)C)C | |
Source | PubChem | |
URL | https://pubchem.ncbi.nlm.nih.gov | |
Description | Data deposited in or computed by PubChem | |
Isomeric SMILES |
CC(=CCC/C(=C/CC/C(=C/CCC(=O)C)/C)/C)C | |
Source | PubChem | |
URL | https://pubchem.ncbi.nlm.nih.gov | |
Description | Data deposited in or computed by PubChem | |
Molecular Formula |
C18H30O | |
Source | PubChem | |
URL | https://pubchem.ncbi.nlm.nih.gov | |
Description | Data deposited in or computed by PubChem | |
DSSTOX Substance ID |
DTXSID5061087, DTXSID50883648 | |
Record name | 5,9,13-Pentadecatrien-2-one, 6,10,14-trimethyl- | |
Source | EPA DSSTox | |
URL | https://comptox.epa.gov/dashboard/DTXSID5061087 | |
Description | DSSTox provides a high quality public chemistry resource for supporting improved predictive toxicology. | |
Record name | 5,9,13-Pentadecatrien-2-one, 6,10,14-trimethyl-, (5E,9E)- | |
Source | EPA DSSTox | |
URL | https://comptox.epa.gov/dashboard/DTXSID50883648 | |
Description | DSSTox provides a high quality public chemistry resource for supporting improved predictive toxicology. | |
Molecular Weight |
262.4 g/mol | |
Source | PubChem | |
URL | https://pubchem.ncbi.nlm.nih.gov | |
Description | Data deposited in or computed by PubChem | |
Physical Description |
Colourless or pale straw-coloured oily liquid; intensely sweet, floral odour | |
Record name | 2,6,10-Trimethyl-2,6,10-pentadecatrien-14-one | |
Source | Joint FAO/WHO Expert Committee on Food Additives (JECFA) | |
URL | https://www.fao.org/food/food-safety-quality/scientific-advice/jecfa/jecfa-flav/details/en/c/1055/ | |
Description | The flavoring agent databse provides the most recent specifications for flavorings evaluated by JECFA. | |
Explanation | Permission from WHO is not required for the use of WHO materials issued under the Creative Commons Attribution-NonCommercial-ShareAlike 3.0 Intergovernmental Organization (CC BY-NC-SA 3.0 IGO) licence. | |
Solubility |
soluble in water; soluble in oils, Miscible at room temperature (in ethanol) | |
Record name | 2,6,10-Trimethyl-2,6,10-pentadecatrien-14-one | |
Source | Joint FAO/WHO Expert Committee on Food Additives (JECFA) | |
URL | https://www.fao.org/food/food-safety-quality/scientific-advice/jecfa/jecfa-flav/details/en/c/1055/ | |
Description | The flavoring agent databse provides the most recent specifications for flavorings evaluated by JECFA. | |
Explanation | Permission from WHO is not required for the use of WHO materials issued under the Creative Commons Attribution-NonCommercial-ShareAlike 3.0 Intergovernmental Organization (CC BY-NC-SA 3.0 IGO) licence. | |
Density |
0.885-0.895 | |
Record name | 2,6,10-Trimethyl-2,6,10-pentadecatrien-14-one | |
Source | Joint FAO/WHO Expert Committee on Food Additives (JECFA) | |
URL | https://www.fao.org/food/food-safety-quality/scientific-advice/jecfa/jecfa-flav/details/en/c/1055/ | |
Description | The flavoring agent databse provides the most recent specifications for flavorings evaluated by JECFA. | |
Explanation | Permission from WHO is not required for the use of WHO materials issued under the Creative Commons Attribution-NonCommercial-ShareAlike 3.0 Intergovernmental Organization (CC BY-NC-SA 3.0 IGO) licence. | |
CAS RN |
1117-52-8, 762-29-8 | |
Record name | Farnesylacetone | |
Source | CAS Common Chemistry | |
URL | https://commonchemistry.cas.org/detail?cas_rn=1117-52-8 | |
Description | CAS Common Chemistry is an open community resource for accessing chemical information. Nearly 500,000 chemical substances from CAS REGISTRY cover areas of community interest, including common and frequently regulated chemicals, and those relevant to high school and undergraduate chemistry classes. This chemical information, curated by our expert scientists, is provided in alignment with our mission as a division of the American Chemical Society. | |
Explanation | The data from CAS Common Chemistry is provided under a CC-BY-NC 4.0 license, unless otherwise stated. | |
Record name | Farnesylacetone | |
Source | ChemIDplus | |
URL | https://pubchem.ncbi.nlm.nih.gov/substance/?source=chemidplus&sourceid=0000762298 | |
Description | ChemIDplus is a free, web search system that provides access to the structure and nomenclature authority files used for the identification of chemical substances cited in National Library of Medicine (NLM) databases, including the TOXNET system. | |
Record name | Farnesyl acetone, (5E,9E)- | |
Source | ChemIDplus | |
URL | https://pubchem.ncbi.nlm.nih.gov/substance/?source=chemidplus&sourceid=0001117528 | |
Description | ChemIDplus is a free, web search system that provides access to the structure and nomenclature authority files used for the identification of chemical substances cited in National Library of Medicine (NLM) databases, including the TOXNET system. | |
Record name | 5,9,13-Pentadecatrien-2-one, 6,10,14-trimethyl-, (5E,9E)- | |
Source | EPA Chemicals under the TSCA | |
URL | https://www.epa.gov/chemicals-under-tsca | |
Description | EPA Chemicals under the Toxic Substances Control Act (TSCA) collection contains information on chemicals and their regulations under TSCA, including non-confidential content from the TSCA Chemical Substance Inventory and Chemical Data Reporting. | |
Record name | 5,9,13-Pentadecatrien-2-one, 6,10,14-trimethyl- | |
Source | EPA Chemicals under the TSCA | |
URL | https://www.epa.gov/chemicals-under-tsca | |
Description | EPA Chemicals under the Toxic Substances Control Act (TSCA) collection contains information on chemicals and their regulations under TSCA, including non-confidential content from the TSCA Chemical Substance Inventory and Chemical Data Reporting. | |
Record name | 5,9,13-Pentadecatrien-2-one, 6,10,14-trimethyl- | |
Source | EPA DSSTox | |
URL | https://comptox.epa.gov/dashboard/DTXSID5061087 | |
Description | DSSTox provides a high quality public chemistry resource for supporting improved predictive toxicology. | |
Record name | 5,9,13-Pentadecatrien-2-one, 6,10,14-trimethyl-, (5E,9E)- | |
Source | EPA DSSTox | |
URL | https://comptox.epa.gov/dashboard/DTXSID50883648 | |
Description | DSSTox provides a high quality public chemistry resource for supporting improved predictive toxicology. | |
Record name | 6,10,14-trimethylpentadeca-5,9,13-trien-2-one | |
Source | European Chemicals Agency (ECHA) | |
URL | https://echa.europa.eu/substance-information/-/substanceinfo/100.010.998 | |
Description | The European Chemicals Agency (ECHA) is an agency of the European Union which is the driving force among regulatory authorities in implementing the EU's groundbreaking chemicals legislation for the benefit of human health and the environment as well as for innovation and competitiveness. | |
Explanation | Use of the information, documents and data from the ECHA website is subject to the terms and conditions of this Legal Notice, and subject to other binding limitations provided for under applicable law, the information, documents and data made available on the ECHA website may be reproduced, distributed and/or used, totally or in part, for non-commercial purposes provided that ECHA is acknowledged as the source: "Source: European Chemicals Agency, http://echa.europa.eu/". Such acknowledgement must be included in each copy of the material. ECHA permits and encourages organisations and individuals to create links to the ECHA website under the following cumulative conditions: Links can only be made to webpages that provide a link to the Legal Notice page. | |
Record name | (E,E)-6,10,14-trimethylpentadeca-5,9,13-trien-2-one | |
Source | European Chemicals Agency (ECHA) | |
URL | https://echa.europa.eu/substance-information/-/substanceinfo/100.012.952 | |
Description | The European Chemicals Agency (ECHA) is an agency of the European Union which is the driving force among regulatory authorities in implementing the EU's groundbreaking chemicals legislation for the benefit of human health and the environment as well as for innovation and competitiveness. | |
Explanation | Use of the information, documents and data from the ECHA website is subject to the terms and conditions of this Legal Notice, and subject to other binding limitations provided for under applicable law, the information, documents and data made available on the ECHA website may be reproduced, distributed and/or used, totally or in part, for non-commercial purposes provided that ECHA is acknowledged as the source: "Source: European Chemicals Agency, http://echa.europa.eu/". Such acknowledgement must be included in each copy of the material. ECHA permits and encourages organisations and individuals to create links to the ECHA website under the following cumulative conditions: Links can only be made to webpages that provide a link to the Legal Notice page. | |
Record name | FARNESYL ACETONE, (5E,9E)- | |
Source | FDA Global Substance Registration System (GSRS) | |
URL | https://gsrs.ncats.nih.gov/ginas/app/beta/substances/3S0G4N267H | |
Description | The FDA Global Substance Registration System (GSRS) enables the efficient and accurate exchange of information on what substances are in regulated products. Instead of relying on names, which vary across regulatory domains, countries, and regions, the GSRS knowledge base makes it possible for substances to be defined by standardized, scientific descriptions. | |
Explanation | Unless otherwise noted, the contents of the FDA website (www.fda.gov), both text and graphics, are not copyrighted. They are in the public domain and may be republished, reprinted and otherwise used freely by anyone without the need to obtain permission from FDA. Credit to the U.S. Food and Drug Administration as the source is appreciated but not required. | |
Retrosynthesis Analysis
AI-Powered Synthesis Planning: Our tool employs the Template_relevance Pistachio, Template_relevance Bkms_metabolic, Template_relevance Pistachio_ringbreaker, Template_relevance Reaxys, Template_relevance Reaxys_biocatalysis model, leveraging a vast database of chemical reactions to predict feasible synthetic routes.
One-Step Synthesis Focus: Specifically designed for one-step synthesis, it provides concise and direct routes for your target compounds, streamlining the synthesis process.
Accurate Predictions: Utilizing the extensive PISTACHIO, BKMS_METABOLIC, PISTACHIO_RINGBREAKER, REAXYS, REAXYS_BIOCATALYSIS database, our tool offers high-accuracy predictions, reflecting the latest in chemical research and data.
Strategy Settings
Precursor scoring | Relevance Heuristic |
---|---|
Min. plausibility | 0.01 |
Model | Template_relevance |
Template Set | Pistachio/Bkms_metabolic/Pistachio_ringbreaker/Reaxys/Reaxys_biocatalysis |
Top-N result to add to graph | 6 |
Feasible Synthetic Routes
Disclaimer and Information on In-Vitro Research Products
Please be aware that all articles and product information presented on BenchChem are intended solely for informational purposes. The products available for purchase on BenchChem are specifically designed for in-vitro studies, which are conducted outside of living organisms. In-vitro studies, derived from the Latin term "in glass," involve experiments performed in controlled laboratory settings using cells or tissues. It is important to note that these products are not categorized as medicines or drugs, and they have not received approval from the FDA for the prevention, treatment, or cure of any medical condition, ailment, or disease. We must emphasize that any form of bodily introduction of these products into humans or animals is strictly prohibited by law. It is essential to adhere to these guidelines to ensure compliance with legal and ethical standards in research and experimentation.