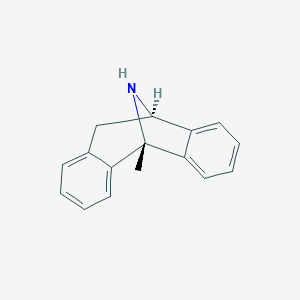
Dizocilpine
Overview
Description
Dizocilpine (MK-801) is a non-competitive antagonist of the N-methyl-D-aspartate (NMDA) receptor, binding to the ion channel site and blocking glutamate-mediated excitatory neurotransmission . It is widely utilized in preclinical research to model schizophrenia due to its ability to induce hyperlocomotion, cognitive deficits, and sensorimotor gating disruptions in rodents . Additionally, this compound exhibits neuroprotective properties in cerebral ischemia models when administered pre- or post-ischemia , and it has shown anticancer activity by inhibiting the ERK1/2 signaling pathway, downregulating cyclin D1, and upregulating tumor suppressors like p21 and p53 .
Preparation Methods
Synthetic Routes and Reaction Conditions: The synthesis of dizocilpine involves several key steps. The nitrogen-bridged core of this compound can be obtained through an intramolecular selective α-Heck reaction followed by an hydroamination reaction between the exocyclic alkene and the secondary protected amine . The reaction conditions typically involve the use of palladium catalysts and specific ligands to facilitate the Heck reaction.
Industrial Production Methods: Industrial production of this compound follows similar synthetic routes but on a larger scale. The process involves optimizing reaction conditions to ensure high yield and purity. This includes controlling temperature, pressure, and the concentration of reactants. The final product is purified using techniques such as crystallization and chromatography.
Chemical Reactions Analysis
Types of Reactions: Dizocilpine undergoes various chemical reactions, including:
Oxidation: this compound can be oxidized under specific conditions to form different oxidation products.
Reduction: Reduction reactions can modify the structure of this compound, potentially altering its pharmacological properties.
Substitution: Substitution reactions can introduce different functional groups into the this compound molecule, affecting its activity and binding affinity.
Common Reagents and Conditions:
Oxidation: Common oxidizing agents include potassium permanganate and chromium trioxide.
Reduction: Reducing agents such as lithium aluminum hydride and sodium borohydride are used.
Substitution: Various halogenating agents and nucleophiles are employed in substitution reactions.
Major Products Formed: The major products formed from these reactions depend on the specific conditions and reagents used. For example, oxidation can lead to the formation of ketones or carboxylic acids, while reduction can yield alcohols or amines.
Scientific Research Applications
Behavioral Research
Dizocilpine has been extensively used in behavioral studies to understand the mechanisms underlying various neuropsychiatric disorders. Its ability to block NMDA receptors makes it a valuable tool for investigating the effects of glutamatergic dysfunction.
Key Findings:
- Cognitive Impairment: Research indicates that this compound can impair cognitive functions, particularly in tasks requiring memory and learning. For instance, studies have shown that chronic administration of this compound can facilitate cocaine self-administration, suggesting its role in addiction behaviors .
- Locomotor Activity: this compound has been shown to induce hyperlocomotion in animal models. In comparative studies, it was found to be approximately 15 times more potent than phencyclidine (PCP) in stimulating locomotion . This effect is dose-dependent, with significant increases observed at doses ranging from 0.1 to 0.2 mg/kg .
Neuroprotective Effects
Recent investigations have highlighted the neuroprotective properties of this compound derivatives. These compounds are being explored as potential treatments for neurodegenerative diseases due to their ability to modulate NMDA receptor activity without inducing psychotomimetic effects.
Research Insights:
- Neuroprotection Against Excitotoxicity: Novel dibenzosuberane derivatives derived from this compound have shown promise in protecting against NMDA-induced hippocampal neurodegeneration. These compounds demonstrated significant reduction in hippocampal damage at concentrations as low as 30 µM .
- Safety Profile: Behavioral studies indicated that certain this compound derivatives did not induce hyperlocomotion or impair startle response in rats, suggesting a favorable safety profile for potential therapeutic applications .
Pharmacological Applications
The pharmacological potential of this compound extends beyond behavioral studies and neuroprotection. It is being investigated for its efficacy in treating various conditions linked to NMDA receptor dysfunction.
Potential Therapeutic Uses:
- Chronic Pain and Depression: Due to its NMDA antagonism, this compound may provide relief in conditions characterized by chronic pain and depressive symptoms by modulating glutamatergic signaling pathways .
- Addiction Treatment: The facilitation of cocaine self-administration through chronic NMDA receptor blockade suggests that this compound could play a role in understanding and potentially treating substance use disorders .
Data Summary Table
Application Area | Description | Key Findings |
---|---|---|
Behavioral Research | Investigating cognitive impairment and locomotor activity | Induces hyperlocomotion; affects learning/memory |
Neuroprotective Effects | Protecting against excitotoxicity | Reduces hippocampal damage; safe derivatives |
Pharmacological Applications | Potential treatment for chronic pain and addiction | Modulates glutamatergic signaling; addiction behavior |
Case Studies
- Cognitive Impairment Study:
- Neuroprotection Case:
- Addiction Behavior Research:
Mechanism of Action
Dizocilpine exerts its effects by binding inside the ion channel of the NMDA receptor, blocking the flow of ions such as calcium through the channel . This blockade is use- and voltage-dependent, meaning the channel must be open for this compound to bind. By inhibiting the NMDA receptor, this compound disrupts synaptic plasticity and neurotransmission, leading to its characteristic effects on memory and learning. Additionally, this compound has been found to act as a nicotinic acetylcholine receptor antagonist and inhibit serotonin and dopamine transporters .
Comparison with Similar Compounds
Comparison with Similar Compounds
NMDA Receptor Antagonists
2.1.1. Phencyclidine (PCP)
- Mechanism: Non-competitive NMDA receptor antagonist.
- Potency : Less potent than dizocilpine in blocking NMDA receptors. In catalepsy models, the minimum effective dose (MED) for PCP was 3.2 mg/kg vs. 0.178 mg/kg for this compound .
- Therapeutic Applications : Used in psychosis research; exacerbates schizophrenia-like behaviors.
- Side Effects : Psychosis, cognitive impairment, and social withdrawal .
2.1.2. Ketamine
- Mechanism: Non-competitive NMDA receptor antagonist with additional opioid and monoaminergic effects.
- Potency : MED for enhancing GHB-induced catalepsy was 17.8 mg/kg, ~100x less potent than this compound .
- Therapeutic Applications : Rapid-acting antidepressant and anesthetic.
- Side Effects : Psychotomimetic effects at high doses; less motor toxicity than this compound .
2.1.3. ADCI
- Mechanism : Structural analog of this compound with NMDA antagonist properties.
- Potency: 10x less potent than this compound in blocking ethanol withdrawal seizures (effective dose range: 1–10 mg/kg vs. This compound’s 0.1–1 mg/kg) .
- Therapeutic Applications: Reduces ethanol withdrawal seizures and tremors.
- Side Effects : Minimal motor incoordination compared to this compound .
2.1.4. FPL12495AA (Remacemide Metabolite)
- Mechanism : NMDA channel blocker with affinity for the this compound-binding site.
- Potency : Inhibits veratridine-induced glutamate release at lower concentrations (12.5 μM) than this compound (100 μM) .
- Side Effects: Limited data, but fewer locomotor disruptions than this compound .
Potassium Channel Blockers
2.2.1. Cyproheptadine and Amitriptyline
- Mechanism : Tricyclic compounds blocking Kv potassium channels.
- Comparison: this compound inhibits both Kv and K_A currents non-selectively (19–28% inhibition at 30 μM) but is less potent than cyproheptadine, which shows higher selectivity for Kv .
- Therapeutic Implications : Cyproheptadine’s central chain enhances Kv selectivity, making it more suitable for channelopathy research than this compound .
Neuroprotective Agents
2.3.1. Minocycline
- Interaction: Attenuates this compound-induced hyperlocomotion and prepulse inhibition (PPI) deficits by normalizing dopamine levels in the frontal cortex and striatum .
- Therapeutic Potential: Demonstrates antipsychotic-like activity, contrasting this compound’s pro-psychotic effects .
Data Tables
Table 1: Comparative Potency of NMDA Antagonists in Behavioral Models
Table 2: Neurochemical Effects in Cancer Models
Contradictions and Model-Dependent Efficacy
- Neuroprotection : this compound reduced cerebral necrosis in rats when administered pre-ischemia but failed to improve hippocampal histopathology in primates post-ischemia .
- Antidepressant Effects : this compound antagonized imipramine’s antidepressant effects in learned helplessness models, highlighting its complex role in mood regulation .
Biological Activity
Dizocilpine, commonly known as MK-801, is a potent non-competitive antagonist of the N-methyl-D-aspartate (NMDA) receptor, primarily recognized for its significant role in neuropharmacology. This compound has been extensively studied for its diverse biological activities, particularly in the context of central nervous system (CNS) disorders. This article provides an in-depth examination of the biological activity of this compound, including its pharmacological properties, mechanisms of action, and therapeutic implications.
Pharmacological Properties
This compound exhibits a wide array of pharmacological effects, including:
- Anticonvulsant Activity : MK-801 has been shown to possess anticonvulsant properties, making it a candidate for treating seizure disorders. Studies indicate that it can effectively reduce seizure activity in various animal models .
- Anesthetic Effects : The drug is also noted for its anesthetic properties, which are attributed to its NMDA receptor antagonism. This characteristic has led to investigations into its potential use in anesthesia .
- Neuroprotective Effects : Recent studies have highlighted this compound's neuroprotective capabilities, particularly in models of neurodegeneration. For instance, it has been shown to reduce hippocampal damage induced by NMDA receptor overstimulation .
The primary mechanism through which this compound exerts its effects is through antagonism of the NMDA receptor. This receptor plays a crucial role in synaptic plasticity and memory function. By blocking this receptor, this compound can influence various neurochemical pathways:
- Oxidative Stress Modulation : MK-801 has been implicated in the formation of oxidative metabolites that may contribute to its biological effects. It generates reactive oxygen species (ROS), which can lead to cellular damage but also play a role in signaling pathways related to neuroprotection .
- Behavioral Effects : Behavioral studies in animal models have demonstrated that this compound can impair working memory and induce anxiety-like behaviors. These effects are likely tied to its impact on glutamatergic signaling pathways .
Research Findings and Case Studies
Several studies have explored the biological activity of this compound through various experimental paradigms:
Case Study: Neuroprotective Effects
In a study examining the neuroprotective effects of this compound, researchers administered the compound to rats subjected to NMDA-induced hippocampal neurodegeneration. Results indicated that this compound significantly mitigated neuronal loss at doses as low as 30 μM, suggesting its potential utility in preventing excitotoxic damage associated with neurological disorders .
Q & A
Basic Research Questions
Q. What experimental models are commonly used to study the neuroprotective effects of dizocilpine, and what methodological parameters are critical for reproducibility?
this compound’s neuroprotective properties are often studied in rodent models of cerebral ischemia. For example, the rat middle cerebral artery occlusion (MCAO) model requires precise monitoring of plasma concentrations (ED50: 0.3 mg/kg) and survival times (e.g., 4-hour post-occlusion) to ensure reproducibility . Methodological rigor includes verifying occlusion success via cerebral blood flow measurements and controlling for variables like body temperature and anesthesia protocols.
Q. How do researchers assess this compound’s selectivity for NMDA receptor subtypes in electrophysiological studies?
Selectivity is evaluated using voltage-clamp techniques on isolated neurons or brain slices. For instance, studies comparing inhibition of potassium currents (Kv and K_A) by this compound enantiomers show non-selective block (e.g., 19–28% inhibition at 30 μM), contrasting with more selective tricyclic compounds like cyproheptadine . Dose-response curves and competitive binding assays with radiolabeled ligands (e.g., [³H]this compound) further validate receptor specificity .
Q. What are standard dosing protocols for this compound in behavioral studies, and how do strain/vendor differences impact outcomes?
this compound is typically administered intraperitoneally at 0.05–0.3 mg/kg to induce NMDA receptor blockade. However, strain differences significantly alter responses. For example, Sprague Dawley rats from Charles River show stronger this compound-induced startle amplitude increases compared to Envigo rats, necessitating vendor-specific dose calibration . Behavioral paradigms (e.g., radial-arm maze for memory errors) must also account for baseline variability in control groups .
Advanced Research Questions
Q. How can contradictory findings on this compound’s neuroprotective vs. neurotoxic effects be reconciled in preclinical studies?
Context-dependent outcomes arise from differences in dosing, timing, and model systems. While this compound reduces ischemia-induced hippocampal damage at 3 mg/kg , prolonged use in neonatal rats (e.g., P7 administration) causes caspase-3-mediated apoptosis and persistent deficits in prefrontal cortex neurons . Researchers must distinguish acute neuroprotection (e.g., ERK pathway inhibition in cancer cells ) from chronic toxicity by integrating histopathology (e.g., Olney’s lesions) and longitudinal behavioral tracking.
Q. What mechanisms underlie this compound’s modulation of ERK1/2 signaling in cancer cells, and how can this inform experimental design?
this compound inhibits ERK1/2 phosphorylation, downregulating CREB-dependent genes (e.g., cyclin D1, bcl-2) in lung adenocarcinoma cells. Methodologically, siRNA silencing (e.g., p21 knockdown) and chromatin immunoprecipitation (ChIP) are used to validate gene targets . Researchers should also assess off-target interactions, such as α7-nicotinic receptor blockade, which may confound results .
Q. What strategies address this compound-induced variability in cognitive assays, such as radial-arm maze performance?
Variability is minimized by standardizing signal intensity (e.g., light brightness in attention tasks) and controlling for this compound’s latency effects (e.g., response delays from 73.8 msec to 102.0 msec) . Factorial designs with covariates (e.g., non-response trial counts) and post hoc tests (e.g., Bonferroni corrections for multiple comparisons) improve statistical robustness .
Q. How do sex and age influence this compound’s behavioral and neurochemical effects in animal models?
Neonatal exposure (P7) in mice reduces parvalbumin interneurons by 50% in adulthood, correlating with anxiety-like behavior . In adult rats, this compound’s startle amplitude effects are sex-dependent, with females showing stronger responses in vendor-specific cohorts . Age- and sex-stratified sampling, combined with immunohistochemistry (e.g., GAD67 labeling), is critical for mechanistic clarity.
Q. What experimental approaches validate this compound’s role in simulating schizophrenia endophenotypes, and what are limitations?
Schizophrenia models use this compound (0.1–0.3 mg/kg) to induce hyperlocomotion, prepulse inhibition (PPI) deficits, and social withdrawal. However, vendor-specific PPI variability (e.g., Charles River vs. Envigo rats) complicates cross-study comparisons . Complementary techniques, such as optogenetic silencing of NMDA receptors in prefrontal cortex, are recommended to isolate this compound-specific effects .
Q. Methodological Considerations Table
Properties
IUPAC Name |
(1S,9R)-1-methyl-16-azatetracyclo[7.6.1.02,7.010,15]hexadeca-2,4,6,10,12,14-hexaene | |
---|---|---|
Source | PubChem | |
URL | https://pubchem.ncbi.nlm.nih.gov | |
Description | Data deposited in or computed by PubChem | |
InChI |
InChI=1S/C16H15N/c1-16-13-8-4-2-6-11(13)10-15(17-16)12-7-3-5-9-14(12)16/h2-9,15,17H,10H2,1H3/t15-,16+/m1/s1 | |
Source | PubChem | |
URL | https://pubchem.ncbi.nlm.nih.gov | |
Description | Data deposited in or computed by PubChem | |
InChI Key |
LBOJYSIDWZQNJS-CVEARBPZSA-N | |
Source | PubChem | |
URL | https://pubchem.ncbi.nlm.nih.gov | |
Description | Data deposited in or computed by PubChem | |
Canonical SMILES |
CC12C3=CC=CC=C3CC(N1)C4=CC=CC=C24 | |
Source | PubChem | |
URL | https://pubchem.ncbi.nlm.nih.gov | |
Description | Data deposited in or computed by PubChem | |
Isomeric SMILES |
C[C@@]12C3=CC=CC=C3C[C@@H](N1)C4=CC=CC=C24 | |
Source | PubChem | |
URL | https://pubchem.ncbi.nlm.nih.gov | |
Description | Data deposited in or computed by PubChem | |
Molecular Formula |
C16H15N | |
Source | PubChem | |
URL | https://pubchem.ncbi.nlm.nih.gov | |
Description | Data deposited in or computed by PubChem | |
Related CAS |
77086-22-7 (Parent) | |
Record name | Dizocilpine [INN] | |
Source | ChemIDplus | |
URL | https://pubchem.ncbi.nlm.nih.gov/substance/?source=chemidplus&sourceid=0077086216 | |
Description | ChemIDplus is a free, web search system that provides access to the structure and nomenclature authority files used for the identification of chemical substances cited in National Library of Medicine (NLM) databases, including the TOXNET system. | |
DSSTOX Substance ID |
DTXSID3048447 | |
Record name | Dizocilpine | |
Source | EPA DSSTox | |
URL | https://comptox.epa.gov/dashboard/DTXSID3048447 | |
Description | DSSTox provides a high quality public chemistry resource for supporting improved predictive toxicology. | |
Molecular Weight |
221.30 g/mol | |
Source | PubChem | |
URL | https://pubchem.ncbi.nlm.nih.gov | |
Description | Data deposited in or computed by PubChem | |
Mechanism of Action |
This study examined the consequences of systemic treatment with either L-dopa or MK-801 on the levels of mRNAs encoding the 65 and 67 kDa isoforms of glutamate decarboxylase (GAD65 and GAD67) in the striatum and globus pallidus (GP) of rats rendered hemiparkinsonian by intranigral 6-hydroxydopamine injection. GADs mRNA levels were assessed by means of in situ hybridization histochemistry. In the striatum, dopamine denervation resulted in increased GAD67 mRNA levels at the rostral and caudal levels, whereas GAD65 showed selective increase at the caudal level. L-dopa and MK-801 treatments showed differential effects on the two GAD isoform levels in rats with 6-hydroxydopamine lesion. The lesion-induced increases in GAD67 transcripts were potentiated by L-dopa but unaffected by MK-801, whereas the increases in GAD65 were suppressed by MK-801 but unaffected by L-dopa. These data suggest a heterogeneity of glutamate-dopamine interaction in the anteroposterior extent of the striatum and show that NMDA-mediated mechanisms are involved in the 6-hydroxydopamine lesion-induced transcriptional changes in striatal GAD65 but not GAD67. In GP, the 6-OHDA lesion elicited increases in both GAD65 and GAD67 mRNA levels. L-dopa or MK-801 treatment suppressed the lesion-induced augmentations in the two GADs mRNA levels. These results indicate that dopamine denervation-induced changes in the functional activity of GP neurons involve both dopamine and glutamate NMDA receptor-mediated mechanisms. Comparison between the effects of L-dopa and MK-801 treatments on markers of the activity of striatal and pallidal GABA neurons further suggest that the impact of these treatments at the GP level do not depend solely on the striatopallidal input. | |
Record name | DIZOCILPINE | |
Source | Hazardous Substances Data Bank (HSDB) | |
URL | https://pubchem.ncbi.nlm.nih.gov/source/hsdb/7641 | |
Description | The Hazardous Substances Data Bank (HSDB) is a toxicology database that focuses on the toxicology of potentially hazardous chemicals. It provides information on human exposure, industrial hygiene, emergency handling procedures, environmental fate, regulatory requirements, nanomaterials, and related areas. The information in HSDB has been assessed by a Scientific Review Panel. | |
Color/Form |
White solid from cyclohexane | |
CAS No. |
77086-21-6 | |
Record name | Dizocilpine | |
Source | CAS Common Chemistry | |
URL | https://commonchemistry.cas.org/detail?cas_rn=77086-21-6 | |
Description | CAS Common Chemistry is an open community resource for accessing chemical information. Nearly 500,000 chemical substances from CAS REGISTRY cover areas of community interest, including common and frequently regulated chemicals, and those relevant to high school and undergraduate chemistry classes. This chemical information, curated by our expert scientists, is provided in alignment with our mission as a division of the American Chemical Society. | |
Explanation | The data from CAS Common Chemistry is provided under a CC-BY-NC 4.0 license, unless otherwise stated. | |
Record name | Dizocilpine [INN] | |
Source | ChemIDplus | |
URL | https://pubchem.ncbi.nlm.nih.gov/substance/?source=chemidplus&sourceid=0077086216 | |
Description | ChemIDplus is a free, web search system that provides access to the structure and nomenclature authority files used for the identification of chemical substances cited in National Library of Medicine (NLM) databases, including the TOXNET system. | |
Record name | Dizocilpine | |
Source | EPA DSSTox | |
URL | https://comptox.epa.gov/dashboard/DTXSID3048447 | |
Description | DSSTox provides a high quality public chemistry resource for supporting improved predictive toxicology. | |
Record name | DIZOCILPINE | |
Source | FDA Global Substance Registration System (GSRS) | |
URL | https://gsrs.ncats.nih.gov/ginas/app/beta/substances/7PY8KH681I | |
Description | The FDA Global Substance Registration System (GSRS) enables the efficient and accurate exchange of information on what substances are in regulated products. Instead of relying on names, which vary across regulatory domains, countries, and regions, the GSRS knowledge base makes it possible for substances to be defined by standardized, scientific descriptions. | |
Explanation | Unless otherwise noted, the contents of the FDA website (www.fda.gov), both text and graphics, are not copyrighted. They are in the public domain and may be republished, reprinted and otherwise used freely by anyone without the need to obtain permission from FDA. Credit to the U.S. Food and Drug Administration as the source is appreciated but not required. | |
Record name | DIZOCILPINE | |
Source | Hazardous Substances Data Bank (HSDB) | |
URL | https://pubchem.ncbi.nlm.nih.gov/source/hsdb/7641 | |
Description | The Hazardous Substances Data Bank (HSDB) is a toxicology database that focuses on the toxicology of potentially hazardous chemicals. It provides information on human exposure, industrial hygiene, emergency handling procedures, environmental fate, regulatory requirements, nanomaterials, and related areas. The information in HSDB has been assessed by a Scientific Review Panel. | |
Melting Point |
68.5-69 °C | |
Record name | DIZOCILPINE | |
Source | Hazardous Substances Data Bank (HSDB) | |
URL | https://pubchem.ncbi.nlm.nih.gov/source/hsdb/7641 | |
Description | The Hazardous Substances Data Bank (HSDB) is a toxicology database that focuses on the toxicology of potentially hazardous chemicals. It provides information on human exposure, industrial hygiene, emergency handling procedures, environmental fate, regulatory requirements, nanomaterials, and related areas. The information in HSDB has been assessed by a Scientific Review Panel. | |
Synthesis routes and methods
Procedure details
Retrosynthesis Analysis
AI-Powered Synthesis Planning: Our tool employs the Template_relevance Pistachio, Template_relevance Bkms_metabolic, Template_relevance Pistachio_ringbreaker, Template_relevance Reaxys, Template_relevance Reaxys_biocatalysis model, leveraging a vast database of chemical reactions to predict feasible synthetic routes.
One-Step Synthesis Focus: Specifically designed for one-step synthesis, it provides concise and direct routes for your target compounds, streamlining the synthesis process.
Accurate Predictions: Utilizing the extensive PISTACHIO, BKMS_METABOLIC, PISTACHIO_RINGBREAKER, REAXYS, REAXYS_BIOCATALYSIS database, our tool offers high-accuracy predictions, reflecting the latest in chemical research and data.
Strategy Settings
Precursor scoring | Relevance Heuristic |
---|---|
Min. plausibility | 0.01 |
Model | Template_relevance |
Template Set | Pistachio/Bkms_metabolic/Pistachio_ringbreaker/Reaxys/Reaxys_biocatalysis |
Top-N result to add to graph | 6 |
Feasible Synthetic Routes
Disclaimer and Information on In-Vitro Research Products
Please be aware that all articles and product information presented on BenchChem are intended solely for informational purposes. The products available for purchase on BenchChem are specifically designed for in-vitro studies, which are conducted outside of living organisms. In-vitro studies, derived from the Latin term "in glass," involve experiments performed in controlled laboratory settings using cells or tissues. It is important to note that these products are not categorized as medicines or drugs, and they have not received approval from the FDA for the prevention, treatment, or cure of any medical condition, ailment, or disease. We must emphasize that any form of bodily introduction of these products into humans or animals is strictly prohibited by law. It is essential to adhere to these guidelines to ensure compliance with legal and ethical standards in research and experimentation.