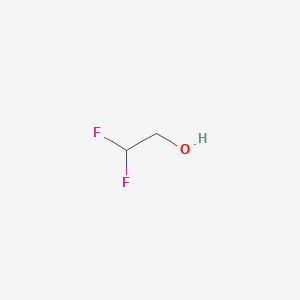
2,2-Difluoroethanol
Overview
Description
2,2-Difluoroethanol (DFE, C₂H₄F₂O) is a fluorinated alcohol with a molecular weight of 82.05 g/mol and a boiling point of 96–97°C . Its structure features two fluorine atoms on the β-carbon, adjacent to the hydroxyl group, which significantly influences its physical and chemical properties. DFE is synthesized via catalytic hydrogenation of difluoroacetyl chloride, a method favored for its simplicity, commercial availability of reagents, and high yield .
DFE exhibits unique thermodynamic properties, including low compressibility compared to other fluorinated alcohols. For instance, at 298.15 K and atmospheric pressure, DFE has a density of 1.252 g/cm³ and an isothermal compressibility of 0.102 GPa⁻¹, lower than 2,2,2-trifluoroethanol (TFE) but higher than 2-fluoroethanol . These properties make it a versatile solvent and intermediate in organic synthesis and materials science.
Scientific Research Applications
Chemical Synthesis
Intermediate for Fluorinated Compounds
2,2-Difluoroethanol is primarily utilized as an intermediate in the synthesis of various fluorinated compounds. It serves as a building block for the production of herbicides, insecticides, and pharmaceutical agents. For instance, it is involved in synthesizing penoxsulam, a widely used herbicide .
Solvent Properties
Due to its polar nature and ability to dissolve a wide range of organic compounds, DFE is often employed as a solvent in chemical reactions. Its use in solvent systems enhances reaction efficiency and product yield. A study demonstrated that DFE can effectively dissolve various substrates in liquid-liquid equilibrium systems .
Pharmaceutical Applications
Medicinal Chemistry
In the pharmaceutical industry, this compound is used to create difluoroethoxy derivatives that have potential therapeutic effects. These derivatives can act as inhibitors for certain enzymes such as mPGE synthetase and PDE-4, which are implicated in inflammatory diseases . The compound's structure allows for modifications that enhance biological activity while maintaining low toxicity profiles.
Case Study: Drug Development
A notable application of DFE in drug development involves its use in synthesizing novel compounds with anti-inflammatory properties. Researchers have reported that difluoroethanol derivatives exhibit significant inhibition of inflammatory pathways, making them candidates for further clinical development .
Material Science
Polymer Synthesis
DFE is also significant in materials science, particularly in the synthesis of fluoropolymers. By incorporating difluoroethanol into polymer matrices, researchers can enhance properties such as thermal stability and chemical resistance. The reaction of DFE with methacrylic acid produces difluoroethyl methacrylate, which can be polymerized to form advanced materials used in coatings and adhesives .
Table: Comparison of Solvents Used with this compound
Solvent Type | Conversion Rate (%) | Reaction Time (h) |
---|---|---|
Tetrahydrofuran (THF) | >99 | 1 |
Glycol Dimethyl Ether | >99 | 1 |
Toluene | >99 | 1 |
This table illustrates the efficiency of different solvents when used with DFE in chemical reactions, highlighting its versatility.
Agricultural Chemicals
Pesticide Formulation
In agriculture, this compound is utilized in formulating pesticides and herbicides due to its effectiveness as a solvent and intermediate. Its role in synthesizing active ingredients for pest control products has been well documented. The compound's ability to enhance the solubility and stability of these formulations contributes to their efficacy in agricultural applications .
Mechanism of Action
The mechanism of action of 2,2-difluoroethanol involves its interaction with various molecular targets and pathways:
Comparison with Similar Compounds
2,2,2-Trifluoroethanol (TFE)
Physical Properties :
- TFE (C₂H₃F₃O) has a higher density (1.384 g/cm³) and lower compressibility than DFE due to the additional fluorine atom .
- Thermodynamic Data :
Property | DFE | TFE |
---|---|---|
Isothermal Compressibility (GPa⁻¹) | 0.102 | 0.085 |
Density (g/cm³, 298 K) | 1.252 | 1.384 |
Chemical Reactivity :
- Acidity : DFE has a pKa of ~12.5, while TFE is more acidic (pKa ~11.5) due to stronger electron-withdrawing effects of the CF₃ group .
- Solvent Efficiency : In Pd/NBE-catalyzed meta-C–H functionalization, DFE outperforms TFE, yielding products in 83% vs. 72% due to better mass balance and coordination effects .
- Bioactivity : CF₂H-containing derivatives (from DFE) often exhibit higher bioactivity than CF₃ analogs (from TFE) in drug candidates, attributed to optimal hydrogen-bonding capabilities .
2-Fluoroethanol
Physical Properties :
- 2-Fluoroethanol (C₂H₅FO) has the lowest compressibility among fluorinated alcohols (0.089 GPa⁻¹ at 298 K) due to reduced fluorine substitution .
Chemical Behavior :
- Acidity: The pKa of 2-fluoroethanol (~14.5) is higher than DFE, reflecting weaker electron-withdrawing effects of a single fluorine atom .
- Synthetic Utility: Unlike DFE, 2-fluoroethanol is less effective in radical transfer reactions, as seen in allylic sulfone functionalization, where DFE achieves 80% yield vs. 45% for 2-fluoroethanol .
Ethanol
Acidity and Reactivity :
- Ethanol (pKa ~16) is far less acidic than DFE (pKa ~12.5), limiting its use in reactions requiring deprotonation .
- Hydrogen Bonding: DFE’s CF₂H group acts as a stronger hydrogen bond donor than ethanol’s CH₃ group, enhancing its role in stabilizing transition states during glycosylation reactions .
Hexafluoroisopropanol (HFIP)
Solvent Effects :
- In Mn-catalyzed C–H oxidation, DFE produces <5% solvent-derived byproducts, whereas HFIP eliminates solvent transfer entirely due to steric hindrance and weak nucleophilicity .
- HFIP’s high polarity (dielectric constant ~16.7) vs. DFE (~12.3) makes it preferable for stabilizing carbocation intermediates in glycosylation .
Pharmaceutical Chemistry
- DFE-derived 2-(2-amino-pyrimidine)-2,2-difluoroethanol scaffolds show promise as type III secretion inhibitors in Gram-negative pathogens, outperforming non-fluorinated analogs .
Battery Electrolytes
- DFE-based electrolytes (e.g., F2DEM) enhance lithium metal battery stability, achieving 98% capacity retention after 200 cycles, compared to 85% for TFE analogs .
Biological Activity
2,2-Difluoroethanol (DFE), with the molecular formula C₂H₄F₂O, is a fluorinated alcohol characterized by its two fluorine atoms attached to the second carbon of the ethyl group. This unique structure imparts distinct chemical properties and biological activities, making it a subject of interest in various fields, including medicinal chemistry and environmental science.
- Molecular Formula : C₂H₄F₂O
- Appearance : Colorless liquid with a distinctive odor
- Boiling Point : 95-96°C
- Solubility : Good solubility in various organic solvents
- Flammability : Highly flammable
The presence of fluorine significantly alters the physical and chemical properties of DFE compared to non-fluorinated alcohols, affecting its reactivity and interactions with biological systems.
Enzyme Interaction and Metabolic Pathways
Research indicates that this compound can influence enzyme activity and metabolic pathways. Its role as a solvent in biochemical reactions allows it to interact with various biological molecules, potentially affecting their function. For instance, studies have shown that DFE can modify the activity of certain enzymes, leading to altered metabolic processes within cells .
Toxicity and Safety
Despite its potential applications, this compound poses certain hazards. It is classified as an acute toxin and an irritant. Inhalation or ingestion can lead to adverse health effects, emphasizing the need for caution when handling this compound . The toxicity profile necessitates further investigation into its safe usage limits in laboratory and industrial settings.
Case Studies and Research Findings
-
Synthesis of Fluorinated Analogs :
A series of 2,2-difluoro-2-arylethylamines were synthesized as analogs of octopamine and noradrenaline. These compounds were designed to explore bioisosteric exchanges where hydroxy groups were replaced by fluorine atoms. Preliminary docking studies indicated varying degrees of compatibility with adrenergic receptors, suggesting potential biological activity similar to natural catecholamines . -
Microbial Degradation Studies :
Research has also focused on the biodegradability of fluorinated compounds like DFE. A study screened Actinobacteria for their ability to degrade fluorinated materials, highlighting the environmental implications of using such compounds . Understanding microbial interactions with DFE can inform strategies for bioremediation in contaminated environments. -
In Silico Studies :
Computational modeling has been employed to predict the behavior of DFE derivatives in biological systems. For example, in silico docking studies assessed the interaction of synthesized fluorinated analogs with β2-adrenergic receptors, providing insights into their potential pharmacological effects .
Comparative Analysis with Similar Compounds
Compound Name | Molecular Formula | Key Features |
---|---|---|
Ethanol | C₂H₅OH | Common alcohol; widely used as a solvent |
1,1-Difluoroethanol | C₂H₄F₂O | Similar structure but different fluorine placement |
1,1,1-Trifluoroethanol | C₂H₃F₃O | Contains three fluorine atoms; higher reactivity |
Trichloroethanol | C₂HCl₃O | Halogenated alcohol; used in industrial applications |
The unique arrangement of fluorine atoms in DFE distinguishes it from other alcohols and fluorinated compounds, impacting its reactivity and biological interactions.
Q & A
Basic Research Questions
Q. What are the key physical and chemical properties of 2,2-difluoroethanol critical for experimental design?
- Answer: this compound (C₂H₄F₂O) is a colorless liquid at room temperature with a molecular weight of 82.05 g/mol. Key properties include a boiling point of 95–97°C, density of 1.296–1.3084 g/cm³, and solubility in water, ethanol, and ethers . Its refractive index (1.3345–1.338) and melting point (-28°C) are critical for solvent selection and low-temperature applications. The compound's stability and fluorination pattern influence its reactivity in organic synthesis .
Q. What safety precautions and handling protocols are essential when working with this compound in laboratory settings?
- Answer: Key precautions include:
- Storage: Keep in a locked, well-ventilated area away from ignition sources .
- PPE: Use chemical-resistant gloves (JIS T 8116), gas masks for organic vapors (JIS T 8152), and safety goggles .
- Emergency measures: Immediate decontamination of skin/eyes with water and consultation with poison control centers if ingested .
- Fire safety: Extinguish using CO₂, dry chemical, or foam .
Q. What are the established synthetic routes for this compound production, and how do their efficiencies compare?
- Answer: Two primary methods are:
- Catalytic hydrogenation of ethyl difluoroacetate: Achieves high yields (>90%) using Cu-Zn-Al-Mn catalysts under hydrogen pressure (5–7 MPa) at 120–150°C .
- Ru/ZrO₂·xH₂O-mediated reduction in water: A greener approach with moderate yields (70–80%), avoiding expensive reagents like NaBH₄. Reaction optimization includes pH control and solvent selection .
Advanced Research Questions
Q. How does fluorination pattern influence the hydrogen bonding network and solvation dynamics of this compound?
- Answer: Computational studies (B3LYP-D3/MP2) show that this compound forms weaker hydrogen bonds compared to non-fluorinated ethanol. The dimer binding energy (ΔE₀) decreases from -5.3 kcal/mol (ethanol) to -4.1 kcal/mol (this compound), attributed to electron-withdrawing fluorine atoms reducing O-H acidity. Vibrational frequency shifts (-Δω) in O-H stretching (∼150 cm⁻¹) confirm altered solvation dynamics .
Q. What mechanistic insights have been gained from copper-catalyzed arylated etherification reactions involving this compound?
- Answer: ESI-MS studies reveal a Cuᴵ intermediate coordinating with this compound and aryl halides (e.g., m-bromobenzotrifluoride). The mechanism involves oxidative addition of the aryl halide to Cuᴵ, followed by nucleophilic attack by the alcohol, forming C-O bonds. Steric effects from fluorine atoms slow down the reaction compared to non-fluorinated analogs .
Q. How does the Brønsted acidity of this compound compare to other fluorinated ethanols in organometallic reactions?
- Answer: this compound has a pKₐ of 13.3 (in water), making it less acidic than 2,2,2-trifluoroethanol (pKₐ 12.5) but more acidic than 2-fluoroethanol (pKₐ 14.4). This acidity enables reversible H₂ splitting with Pdᴵᴵ pincer complexes, favoring alkoxide formation in equilibrium studies .
Q. What analytical challenges exist in characterizing reaction intermediates during this compound-based syntheses?
- Answer: Challenges include:
- Transient intermediates: Short-lived Cuᴵ or Pdᴵᴵ species require time-resolved techniques like stopped-flow spectroscopy.
- Fluorine interference: ¹⁹F NMR is essential but complicated by coupling with adjacent protons. High-resolution MS (ESI-TOF) is preferred for identifying metal-alcohol adducts .
Q. How do environmental factors like atmospheric half-life and aqueous solubility impact the ecological risk assessment of this compound?
- Answer: Atmospheric modeling shows a moderate half-life of 7.0–15.2 days due to OH radical oxidation. Its solubility (0.04–0.15 mol/L) suggests moderate mobility in aquatic systems, requiring biodegradation studies to assess bioaccumulation risks .
Methodological Notes
- Synthesis optimization: Catalyst recycling in hydrogenation reactions improves cost-efficiency (e.g., Cu-Zn-Al-Mn catalysts retain >85% activity after 5 cycles) .
- Computational validation: Compare DFT-derived vibrational frequencies with experimental IR/Raman data to refine solvation models .
- Safety protocols: Regular PPE integrity checks and static discharge controls are critical due to low flash points (3°C) .
Properties
IUPAC Name |
2,2-difluoroethanol | |
---|---|---|
Source | PubChem | |
URL | https://pubchem.ncbi.nlm.nih.gov | |
Description | Data deposited in or computed by PubChem | |
InChI |
InChI=1S/C2H4F2O/c3-2(4)1-5/h2,5H,1H2 | |
Source | PubChem | |
URL | https://pubchem.ncbi.nlm.nih.gov | |
Description | Data deposited in or computed by PubChem | |
InChI Key |
VOGSDFLJZPNWHY-UHFFFAOYSA-N | |
Source | PubChem | |
URL | https://pubchem.ncbi.nlm.nih.gov | |
Description | Data deposited in or computed by PubChem | |
Canonical SMILES |
C(C(F)F)O | |
Source | PubChem | |
URL | https://pubchem.ncbi.nlm.nih.gov | |
Description | Data deposited in or computed by PubChem | |
Molecular Formula |
C2H4F2O | |
Source | PubChem | |
URL | https://pubchem.ncbi.nlm.nih.gov | |
Description | Data deposited in or computed by PubChem | |
DSSTOX Substance ID |
DTXSID90189459 | |
Record name | 2,2-Difluoroethanol | |
Source | EPA DSSTox | |
URL | https://comptox.epa.gov/dashboard/DTXSID90189459 | |
Description | DSSTox provides a high quality public chemistry resource for supporting improved predictive toxicology. | |
Molecular Weight |
82.05 g/mol | |
Source | PubChem | |
URL | https://pubchem.ncbi.nlm.nih.gov | |
Description | Data deposited in or computed by PubChem | |
CAS No. |
359-13-7 | |
Record name | Difluoroethanol | |
Source | ChemIDplus | |
URL | https://pubchem.ncbi.nlm.nih.gov/substance/?source=chemidplus&sourceid=0000359137 | |
Description | ChemIDplus is a free, web search system that provides access to the structure and nomenclature authority files used for the identification of chemical substances cited in National Library of Medicine (NLM) databases, including the TOXNET system. | |
Record name | 2,2-Difluoroethanol | |
Source | EPA DSSTox | |
URL | https://comptox.epa.gov/dashboard/DTXSID90189459 | |
Description | DSSTox provides a high quality public chemistry resource for supporting improved predictive toxicology. | |
Record name | 2,2-Difluoroethanol | |
Source | European Chemicals Agency (ECHA) | |
URL | https://echa.europa.eu/information-on-chemicals | |
Description | The European Chemicals Agency (ECHA) is an agency of the European Union which is the driving force among regulatory authorities in implementing the EU's groundbreaking chemicals legislation for the benefit of human health and the environment as well as for innovation and competitiveness. | |
Explanation | Use of the information, documents and data from the ECHA website is subject to the terms and conditions of this Legal Notice, and subject to other binding limitations provided for under applicable law, the information, documents and data made available on the ECHA website may be reproduced, distributed and/or used, totally or in part, for non-commercial purposes provided that ECHA is acknowledged as the source: "Source: European Chemicals Agency, http://echa.europa.eu/". Such acknowledgement must be included in each copy of the material. ECHA permits and encourages organisations and individuals to create links to the ECHA website under the following cumulative conditions: Links can only be made to webpages that provide a link to the Legal Notice page. | |
Retrosynthesis Analysis
AI-Powered Synthesis Planning: Our tool employs the Template_relevance Pistachio, Template_relevance Bkms_metabolic, Template_relevance Pistachio_ringbreaker, Template_relevance Reaxys, Template_relevance Reaxys_biocatalysis model, leveraging a vast database of chemical reactions to predict feasible synthetic routes.
One-Step Synthesis Focus: Specifically designed for one-step synthesis, it provides concise and direct routes for your target compounds, streamlining the synthesis process.
Accurate Predictions: Utilizing the extensive PISTACHIO, BKMS_METABOLIC, PISTACHIO_RINGBREAKER, REAXYS, REAXYS_BIOCATALYSIS database, our tool offers high-accuracy predictions, reflecting the latest in chemical research and data.
Strategy Settings
Precursor scoring | Relevance Heuristic |
---|---|
Min. plausibility | 0.01 |
Model | Template_relevance |
Template Set | Pistachio/Bkms_metabolic/Pistachio_ringbreaker/Reaxys/Reaxys_biocatalysis |
Top-N result to add to graph | 6 |
Feasible Synthetic Routes
Disclaimer and Information on In-Vitro Research Products
Please be aware that all articles and product information presented on BenchChem are intended solely for informational purposes. The products available for purchase on BenchChem are specifically designed for in-vitro studies, which are conducted outside of living organisms. In-vitro studies, derived from the Latin term "in glass," involve experiments performed in controlled laboratory settings using cells or tissues. It is important to note that these products are not categorized as medicines or drugs, and they have not received approval from the FDA for the prevention, treatment, or cure of any medical condition, ailment, or disease. We must emphasize that any form of bodily introduction of these products into humans or animals is strictly prohibited by law. It is essential to adhere to these guidelines to ensure compliance with legal and ethical standards in research and experimentation.