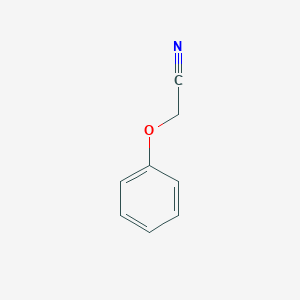
Phenoxyacetonitrile
Overview
Description
Phenoxyacetonitrile, also known as 2-phenoxyacetonitrile, is an organic compound with the molecular formula C8H7NO. It is a colorless to almost colorless liquid with a faint aromatic odor. This compound is used as an intermediate in organic synthesis and has applications in various fields, including pharmaceuticals and agrochemicals .
Preparation Methods
Synthetic Routes and Reaction Conditions: Phenoxyacetonitrile can be synthesized through several methods. One common method involves the reaction of phenol with chloroacetonitrile in the presence of a base such as sodium hydroxide. The reaction proceeds via nucleophilic substitution, where the phenoxide ion attacks the carbon atom of chloroacetonitrile, resulting in the formation of this compound .
Industrial Production Methods: In industrial settings, this compound is typically produced through similar nucleophilic substitution reactions but on a larger scale. The reaction conditions are optimized to ensure high yield and purity of the product. The process involves careful control of temperature, pressure, and the use of catalysts to enhance the reaction rate .
Chemical Reactions Analysis
Types of Reactions: Phenoxyacetonitrile undergoes various chemical reactions, including:
Oxidation: this compound can be oxidized to form phenoxyacetic acid using oxidizing agents such as potassium permanganate or hydrogen peroxide.
Reduction: Reduction of this compound can yield phenoxyethylamine using reducing agents like lithium aluminum hydride.
Common Reagents and Conditions:
Oxidation: Potassium permanganate, hydrogen peroxide, acidic or basic conditions.
Reduction: Lithium aluminum hydride, sodium borohydride, catalytic hydrogenation.
Substitution: Bases like sodium hydroxide, catalysts like palladium on carbon.
Major Products Formed:
Oxidation: Phenoxyacetic acid.
Reduction: Phenoxyethylamine.
Substitution: Various substituted this compound derivatives.
Scientific Research Applications
Phenoxyacetonitrile has several applications in scientific research:
Chemistry: It is used as a building block in the synthesis of various organic compounds, including pharmaceuticals and agrochemicals.
Biology: this compound derivatives have been studied for their potential biological activities, including antimicrobial and anticancer properties.
Medicine: It serves as an intermediate in the synthesis of drugs and other bioactive molecules.
Industry: this compound is used in the production of dyes, pigments, and other industrial chemicals.
Mechanism of Action
The mechanism of action of phenoxyacetonitrile involves its ability to undergo various chemical transformations, making it a versatile intermediate in organic synthesis. The nitrile group in this compound can participate in nucleophilic addition reactions, forming imines or amines, which can further react to form a wide range of compounds. The phenoxy group provides stability and can influence the reactivity of the nitrile group .
Comparison with Similar Compounds
Phenoxyacetonitrile can be compared with other similar compounds such as:
Benzyl cyanide (C8H7N): Similar structure but lacks the phenoxy group, making it less reactive in certain nucleophilic substitution reactions.
Phenylacetonitrile (C8H7N): Similar to benzyl cyanide but with a different substitution pattern, affecting its reactivity and applications.
4-Methoxybenzonitrile (C8H7NO): Contains a methoxy group instead of a phenoxy group, leading to different chemical properties and reactivity.
This compound is unique due to the presence of both the phenoxy and nitrile groups, which provide a balance of stability and reactivity, making it a valuable intermediate in organic synthesis.
Properties
IUPAC Name |
2-phenoxyacetonitrile | |
---|---|---|
Source | PubChem | |
URL | https://pubchem.ncbi.nlm.nih.gov | |
Description | Data deposited in or computed by PubChem | |
InChI |
InChI=1S/C8H7NO/c9-6-7-10-8-4-2-1-3-5-8/h1-5H,7H2 | |
Source | PubChem | |
URL | https://pubchem.ncbi.nlm.nih.gov | |
Description | Data deposited in or computed by PubChem | |
InChI Key |
VLLSCJFPVSQXDM-UHFFFAOYSA-N | |
Source | PubChem | |
URL | https://pubchem.ncbi.nlm.nih.gov | |
Description | Data deposited in or computed by PubChem | |
Canonical SMILES |
C1=CC=C(C=C1)OCC#N | |
Source | PubChem | |
URL | https://pubchem.ncbi.nlm.nih.gov | |
Description | Data deposited in or computed by PubChem | |
Molecular Formula |
C8H7NO | |
Source | PubChem | |
URL | https://pubchem.ncbi.nlm.nih.gov | |
Description | Data deposited in or computed by PubChem | |
DSSTOX Substance ID |
DTXSID60287158 | |
Record name | Phenoxyacetonitrile | |
Source | EPA DSSTox | |
URL | https://comptox.epa.gov/dashboard/DTXSID60287158 | |
Description | DSSTox provides a high quality public chemistry resource for supporting improved predictive toxicology. | |
Molecular Weight |
133.15 g/mol | |
Source | PubChem | |
URL | https://pubchem.ncbi.nlm.nih.gov | |
Description | Data deposited in or computed by PubChem | |
CAS No. |
3598-14-9 | |
Record name | 2-Phenoxyacetonitrile | |
Source | ChemIDplus | |
URL | https://pubchem.ncbi.nlm.nih.gov/substance/?source=chemidplus&sourceid=0003598149 | |
Description | ChemIDplus is a free, web search system that provides access to the structure and nomenclature authority files used for the identification of chemical substances cited in National Library of Medicine (NLM) databases, including the TOXNET system. | |
Record name | 3598-14-9 | |
Source | DTP/NCI | |
URL | https://dtp.cancer.gov/dtpstandard/servlet/dwindex?searchtype=NSC&outputformat=html&searchlist=49354 | |
Description | The NCI Development Therapeutics Program (DTP) provides services and resources to the academic and private-sector research communities worldwide to facilitate the discovery and development of new cancer therapeutic agents. | |
Explanation | Unless otherwise indicated, all text within NCI products is free of copyright and may be reused without our permission. Credit the National Cancer Institute as the source. | |
Record name | Phenoxyacetonitrile | |
Source | EPA DSSTox | |
URL | https://comptox.epa.gov/dashboard/DTXSID60287158 | |
Description | DSSTox provides a high quality public chemistry resource for supporting improved predictive toxicology. | |
Record name | Phenoxyacetonitrile | |
Source | European Chemicals Agency (ECHA) | |
URL | https://echa.europa.eu/information-on-chemicals | |
Description | The European Chemicals Agency (ECHA) is an agency of the European Union which is the driving force among regulatory authorities in implementing the EU's groundbreaking chemicals legislation for the benefit of human health and the environment as well as for innovation and competitiveness. | |
Explanation | Use of the information, documents and data from the ECHA website is subject to the terms and conditions of this Legal Notice, and subject to other binding limitations provided for under applicable law, the information, documents and data made available on the ECHA website may be reproduced, distributed and/or used, totally or in part, for non-commercial purposes provided that ECHA is acknowledged as the source: "Source: European Chemicals Agency, http://echa.europa.eu/". Such acknowledgement must be included in each copy of the material. ECHA permits and encourages organisations and individuals to create links to the ECHA website under the following cumulative conditions: Links can only be made to webpages that provide a link to the Legal Notice page. | |
Record name | 2-Phenoxyacetonitrile | |
Source | FDA Global Substance Registration System (GSRS) | |
URL | https://gsrs.ncats.nih.gov/ginas/app/beta/substances/QU78TF2FK8 | |
Description | The FDA Global Substance Registration System (GSRS) enables the efficient and accurate exchange of information on what substances are in regulated products. Instead of relying on names, which vary across regulatory domains, countries, and regions, the GSRS knowledge base makes it possible for substances to be defined by standardized, scientific descriptions. | |
Explanation | Unless otherwise noted, the contents of the FDA website (www.fda.gov), both text and graphics, are not copyrighted. They are in the public domain and may be republished, reprinted and otherwise used freely by anyone without the need to obtain permission from FDA. Credit to the U.S. Food and Drug Administration as the source is appreciated but not required. | |
Retrosynthesis Analysis
AI-Powered Synthesis Planning: Our tool employs the Template_relevance Pistachio, Template_relevance Bkms_metabolic, Template_relevance Pistachio_ringbreaker, Template_relevance Reaxys, Template_relevance Reaxys_biocatalysis model, leveraging a vast database of chemical reactions to predict feasible synthetic routes.
One-Step Synthesis Focus: Specifically designed for one-step synthesis, it provides concise and direct routes for your target compounds, streamlining the synthesis process.
Accurate Predictions: Utilizing the extensive PISTACHIO, BKMS_METABOLIC, PISTACHIO_RINGBREAKER, REAXYS, REAXYS_BIOCATALYSIS database, our tool offers high-accuracy predictions, reflecting the latest in chemical research and data.
Strategy Settings
Precursor scoring | Relevance Heuristic |
---|---|
Min. plausibility | 0.01 |
Model | Template_relevance |
Template Set | Pistachio/Bkms_metabolic/Pistachio_ringbreaker/Reaxys/Reaxys_biocatalysis |
Top-N result to add to graph | 6 |
Feasible Synthetic Routes
Q1: What are the common synthetic routes to benzofurans starting from phenoxyacetonitrile?
A: Two main synthetic approaches utilize this compound as a precursor to benzofurans. The first method employs a Vilsmeier reaction, where this compound reacts with a Vilsmeier reagent (typically generated from phosphorus oxychloride and dimethylformamide) to yield 2-benzofurancarboxylic acid derivatives []. Alternatively, a palladium-catalyzed approach involves the addition of arylboronic acids to this compound in the presence of a cationic palladium catalyst, leading to the formation of benzofurans in a single step [, ].
Q2: Can this compound be used to synthesize other heterocycles besides benzofurans?
A: Yes, this compound acts as a valuable synthon for a range of heterocycles. For instance, it can undergo vicarious nucleophilic substitution (VNS) reactions with nitro(pentafluorosulfanyl)benzenes, leading to the formation of pentafluorosulfanyl-containing indoles and oxindoles after subsequent modifications [].
Q3: How does the structure of the aryloxy group in aryloxyacetonitriles affect their reactivity in light-induced rearrangements?
A: Research shows that various aryloxyacetonitriles, including those with substituents like methyl or methoxy groups on the aromatic ring, undergo similar light-induced rearrangements in methanol []. This suggests that the electronic nature of the substituent on the aryloxy group doesn't drastically alter the reaction pathway.
Q4: Has this compound been utilized in studies investigating biological targets?
A: While this compound itself might not be the final biologically active compound, its derivatives have shown potential in targeting specific interactions. For instance, studies investigating the carboxylate-imidazolium hydrogen bond, crucial in biological systems like the aspartate-histidine couple in serine proteases, utilize this compound derivatives [].
Disclaimer and Information on In-Vitro Research Products
Please be aware that all articles and product information presented on BenchChem are intended solely for informational purposes. The products available for purchase on BenchChem are specifically designed for in-vitro studies, which are conducted outside of living organisms. In-vitro studies, derived from the Latin term "in glass," involve experiments performed in controlled laboratory settings using cells or tissues. It is important to note that these products are not categorized as medicines or drugs, and they have not received approval from the FDA for the prevention, treatment, or cure of any medical condition, ailment, or disease. We must emphasize that any form of bodily introduction of these products into humans or animals is strictly prohibited by law. It is essential to adhere to these guidelines to ensure compliance with legal and ethical standards in research and experimentation.