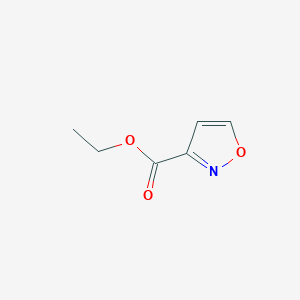
Ethyl isoxazole-3-carboxylate
Overview
Description
Ethyl isoxazole-3-carboxylate is a heterocyclic compound featuring an isoxazole ring, which is a five-membered ring containing one oxygen and one nitrogen atom at adjacent positions
Mechanism of Action
Target of Action
Isoxazole derivatives have been known to bind to various biological targets based on their chemical diversity .
Mode of Action
Isoxazole derivatives are known to interact with their targets in a variety of ways, often leading to significant changes in cellular processes . The interaction of Ethyl isoxazole-3-carboxylate with its targets would likely depend on the specific chemical structure of the compound and the nature of the target.
Biochemical Pathways
Isoxazole derivatives are known to influence a wide range of biochemical pathways, often resulting in diverse downstream effects . The exact pathways affected by this compound would likely depend on its specific targets and mode of action.
Result of Action
Isoxazole derivatives are known to exert a wide range of biological activities, including antimicrobial, antiviral, antitumor, anti-inflammatory, immunomodulating, anticonvulsant, antidiabetic, etc . The specific effects of this compound would likely depend on its specific targets and mode of action.
Preparation Methods
Synthetic Routes and Reaction Conditions: Ethyl isoxazole-3-carboxylate can be synthesized through various methods. One common approach involves the reaction of ethyl nitroacetate with phenylacetylene in the presence of a catalyst such as TEMPO, water, and open air, yielding 3,5-disubstituted isoxazole . Another method involves the cyclization of ethyl-2-chloro-2-(hydroxyimino)acetate with dipolarophiles under microwave conditions .
Industrial Production Methods: Industrial production of this compound typically involves large-scale synthesis using optimized reaction conditions to ensure high yield and purity. The use of eco-friendly and cost-effective catalysts is preferred to minimize environmental impact and production costs.
Chemical Reactions Analysis
Types of Reactions: Ethyl isoxazole-3-carboxylate undergoes various chemical reactions, including:
Oxidation: The compound can be oxidized to form different derivatives.
Reduction: Reduction reactions can modify the functional groups attached to the isoxazole ring.
Substitution: Substitution reactions can introduce new functional groups into the molecule.
Common Reagents and Conditions:
Oxidation: Common oxidizing agents include potassium permanganate and hydrogen peroxide.
Reduction: Reducing agents such as lithium aluminum hydride and sodium borohydride are used.
Substitution: Reagents like alkyl halides and acyl chlorides are commonly employed.
Major Products Formed: The major products formed from these reactions depend on the specific reagents and conditions used. For example, oxidation may yield carboxylic acids, while reduction can produce alcohols or amines.
Scientific Research Applications
Ethyl isoxazole-3-carboxylate has a wide range of applications in scientific research:
Chemistry: It is used as a building block in the synthesis of more complex molecules.
Medicine: It serves as a precursor in the development of pharmaceutical agents with therapeutic potential.
Industry: The compound is utilized in the production of agrochemicals and other industrial products.
Comparison with Similar Compounds
Ethyl isoxazole-3-carboxylate can be compared with other similar compounds, such as:
Isoxazole: The parent compound with a similar ring structure but without the ethyl carboxylate group.
Mthis compound: A similar compound with a methyl group instead of an ethyl group.
Isoxazole-5-carboxylate: A compound with the carboxylate group at a different position on the ring.
Uniqueness: this compound is unique due to its specific substitution pattern, which imparts distinct chemical and biological properties. Its ethyl carboxylate group enhances its solubility and reactivity compared to other isoxazole derivatives .
Properties
IUPAC Name |
ethyl 1,2-oxazole-3-carboxylate | |
---|---|---|
Source | PubChem | |
URL | https://pubchem.ncbi.nlm.nih.gov | |
Description | Data deposited in or computed by PubChem | |
InChI |
InChI=1S/C6H7NO3/c1-2-9-6(8)5-3-4-10-7-5/h3-4H,2H2,1H3 | |
Source | PubChem | |
URL | https://pubchem.ncbi.nlm.nih.gov | |
Description | Data deposited in or computed by PubChem | |
InChI Key |
RKXWKTOBQOSONL-UHFFFAOYSA-N | |
Source | PubChem | |
URL | https://pubchem.ncbi.nlm.nih.gov | |
Description | Data deposited in or computed by PubChem | |
Canonical SMILES |
CCOC(=O)C1=NOC=C1 | |
Source | PubChem | |
URL | https://pubchem.ncbi.nlm.nih.gov | |
Description | Data deposited in or computed by PubChem | |
Molecular Formula |
C6H7NO3 | |
Source | PubChem | |
URL | https://pubchem.ncbi.nlm.nih.gov | |
Description | Data deposited in or computed by PubChem | |
DSSTOX Substance ID |
DTXSID20434429 | |
Record name | ethyl isoxazole-3-carboxylate | |
Source | EPA DSSTox | |
URL | https://comptox.epa.gov/dashboard/DTXSID20434429 | |
Description | DSSTox provides a high quality public chemistry resource for supporting improved predictive toxicology. | |
Molecular Weight |
141.12 g/mol | |
Source | PubChem | |
URL | https://pubchem.ncbi.nlm.nih.gov | |
Description | Data deposited in or computed by PubChem | |
CAS No. |
3209-70-9 | |
Record name | ethyl isoxazole-3-carboxylate | |
Source | EPA DSSTox | |
URL | https://comptox.epa.gov/dashboard/DTXSID20434429 | |
Description | DSSTox provides a high quality public chemistry resource for supporting improved predictive toxicology. | |
Synthesis routes and methods
Procedure details
Retrosynthesis Analysis
AI-Powered Synthesis Planning: Our tool employs the Template_relevance Pistachio, Template_relevance Bkms_metabolic, Template_relevance Pistachio_ringbreaker, Template_relevance Reaxys, Template_relevance Reaxys_biocatalysis model, leveraging a vast database of chemical reactions to predict feasible synthetic routes.
One-Step Synthesis Focus: Specifically designed for one-step synthesis, it provides concise and direct routes for your target compounds, streamlining the synthesis process.
Accurate Predictions: Utilizing the extensive PISTACHIO, BKMS_METABOLIC, PISTACHIO_RINGBREAKER, REAXYS, REAXYS_BIOCATALYSIS database, our tool offers high-accuracy predictions, reflecting the latest in chemical research and data.
Strategy Settings
Precursor scoring | Relevance Heuristic |
---|---|
Min. plausibility | 0.01 |
Model | Template_relevance |
Template Set | Pistachio/Bkms_metabolic/Pistachio_ringbreaker/Reaxys/Reaxys_biocatalysis |
Top-N result to add to graph | 6 |
Feasible Synthetic Routes
Disclaimer and Information on In-Vitro Research Products
Please be aware that all articles and product information presented on BenchChem are intended solely for informational purposes. The products available for purchase on BenchChem are specifically designed for in-vitro studies, which are conducted outside of living organisms. In-vitro studies, derived from the Latin term "in glass," involve experiments performed in controlled laboratory settings using cells or tissues. It is important to note that these products are not categorized as medicines or drugs, and they have not received approval from the FDA for the prevention, treatment, or cure of any medical condition, ailment, or disease. We must emphasize that any form of bodily introduction of these products into humans or animals is strictly prohibited by law. It is essential to adhere to these guidelines to ensure compliance with legal and ethical standards in research and experimentation.