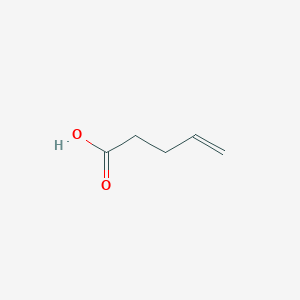
4-Pentenoic acid
Overview
Description
4-Pentenoic acid (4-PA) is a short-chain unsaturated carboxylic acid (CH₂=CHCH₂CH₂COOH) with a terminal double bond. It is structurally analogous to methylenecyclopropylacetic acid, a metabolite of hypoglycin A implicated in Jamaican vomiting sickness . 4-PA has been widely studied for its metabolic effects, particularly its role in inhibiting fatty acid β-oxidation and urea cycle enzymes, leading to hyperammonemia, hypoglycemia, and microvesicular steatosis in animal models . It is also a key experimental tool for modeling Reye’s syndrome and investigating drug-induced hepatotoxicity . Additionally, 4-PA serves as a precursor in organic synthesis, enabling applications in crosslinking polymers (e.g., cellulose derivatives) and catalytic reactions (e.g., Pd-catalyzed amination) .
Preparation Methods
Catalytic Rearrangement of Propylene Alcohol and Orthoacetate
Reaction Mechanism and Conditions
The method described in patent CN101157608A utilizes propylene alcohol (allyl alcohol) and orthoacetate (e.g., trimethyl orthoacetate) as starting materials . The reaction proceeds via transesterification and Claisen rearrangement under acidic or Lewis acid catalysis (Figure 1).
Step 1: Transesterification and Rearrangement
Propylene alcohol reacts with orthoacetate at 60–200°C (optimized: 80–150°C) in the presence of catalysts such as phosphoric acid, aluminum chloride, or propionic acid. The reaction generates 4-pentenoate esters (e.g., methyl 4-pentenoate) through a -sigmatropic shift.
Step 2: Hydrolysis and Acidification
The ester undergoes alkaline hydrolysis (5–50% NaOH/KOH, 50–100°C) followed by acidification (H2SO4/HCl) to yield 4-PA. This step achieves >98% purity and 87–92% yield .
Catalytic Systems and Optimization
Catalyst | Temperature (°C) | Reaction Time (h) | Yield (%) |
---|---|---|---|
Phosphoric acid | 135 | 8 | 87.1 |
Aluminum chloride | 150 | 9 | 92.0 |
Propionic acid | 150 | 7 | 90.2 |
Key Advantages :
-
Scalable to industrial production with minimal byproducts.
Wittig Olefination for Substituted 4-Pentenoic Acids
Synthesis of 5-Phenyl-4-Pentenoic Acid
The Wittig reaction, as demonstrated in ChemicalBook’s protocol, enables the synthesis of 5-phenyl-4-pentenoic acid (yield: 95%) .
Reaction Scheme :
-
Ylide Formation : (3-Carboxypropyl)triphenylphosphonium bromide reacts with sodium hexamethyldisilazane (NaHMDS) in THF at 0°C.
-
Olefination : Benzaldehyde is added at -78°C, followed by warming to 20°C to form the α,β-unsaturated acid.
Conditions :
-
Solvent: Tetrahydrofuran (THF).
-
Temperature: -78°C to 20°C.
-
Workup: Acidification (HCl) and extraction with ethyl acetate.
Scope and Limitations
-
Substituent Compatibility : Effective for aryl and alkyl aldehydes.
-
Drawbacks : Requires stoichiometric phosphonium reagents, increasing cost and waste.
Green Synthesis from Gamma-Valerolactone (GVL)
Catalytic Conversion Over SiO2/Al2O3
Recent advancements highlight the conversion of lignocellulose-derived GVL to 4-PA using SiO2/Al2O3 catalysts .
Mechanism :
-
Ring-Opening : GVL undergoes acid-catalyzed ring-opening to form pentenoic acid.
-
Isomerization : Lewis acid sites on SiO2/Al2O3 promote double-bond migration to the 4-position.
Optimized Conditions :
Industrial Relevance
-
Sustainability : Utilizes renewable biomass feedstocks.
-
Cost-Efficiency : Avoids noble metal catalysts.
Allyl Chloride–Diethyl Malonate Condensation
Alkylation and Decarboxylation
Patent CN101200425A outlines a two-step synthesis from allyl chloride and diethyl malonate :
Step 1: Alkylation
Diethyl malonate reacts with allyl chloride under alkaline conditions (NaOEt/NaOMe, 20–40°C, 2–4 h) to form 2-allyl diethyl malonate .
Step 2: Saponification and Decarboxylation
-
Hydrolysis : 2-allyl malonate ester is saponified with NaOH.
-
Decarboxylation : Heating induces CO2 elimination, yielding 4-PA.
Yield : >85% (overall).
Advantages Over Traditional Methods
-
Low-Temperature Operation : Reduces side reactions.
-
Cost-Effective Reagents : Allyl chloride is inexpensive and widely available.
Comparative Analysis of Methods
Method | Starting Materials | Catalyst | Yield (%) | Selectivity (%) | Scalability |
---|---|---|---|---|---|
Catalytic Rearrangement | Allyl alcohol, orthoacetate | H3PO4, AlCl3 | 87–92 | >98 | High |
Wittig Olefination | Phosphonium salt, aldehyde | NaHMDS | 95 | 90–95 | Low |
GVL Conversion | Gamma-valerolactone | SiO2/Al2O3 | 91 | 98 | Moderate |
Allyl Chloride Condensation | Allyl chloride, diethyl malonate | NaOEt | >85 | 90 | High |
Key Insights :
-
Catalytic Rearrangement and Allyl Chloride Condensation are optimal for bulk production due to high scalability and yields.
-
GVL Conversion offers ecological benefits but requires further optimization for energy-intensive steps.
-
Wittig Olefination remains niche for specialized derivatives.
Chemical Reactions Analysis
Types of Reactions: 4-Pentenoic acid undergoes various chemical reactions, including:
Oxidation: It can be oxidized to form corresponding lactones.
Reduction: It can be reduced to form saturated carboxylic acids.
Substitution: It can participate in substitution reactions to form various derivatives
Common Reagents and Conditions:
Oxidation: Common oxidizing agents include potassium permanganate and chromium trioxide.
Reduction: Hydrogen gas in the presence of a palladium catalyst is often used.
Substitution: Halogenating agents such as bromine can be used for substitution reactions.
Major Products:
Oxidation: γ-lactones.
Reduction: Saturated carboxylic acids.
Substitution: Halogenated derivatives.
Scientific Research Applications
Biochemical Studies
4-Pentenoic acid has been utilized in biochemical research to study fatty acid oxidation. A study demonstrated its effectiveness in inhibiting fatty acid oxidation in rat heart mitochondria, providing insights into metabolic pathways and potential therapeutic targets for metabolic disorders .
Chemoenzymatic Reactions
Recent advancements have highlighted the role of this compound in chemoenzymatic halocyclization processes. Research scaled up the bromolactonization of this compound from laboratory to preparative scales, revealing significant insights into enzyme inhibition and substrate concentration effects. This work emphasizes the compound's utility in developing environmentally friendly synthetic methodologies .
Polyhydroxyalkanoate Production
This compound serves as a precursor for poly(3-hydroxy-4-pentenoic acid) (P(HPE)), a type of polyhydroxyalkanoate (PHA). PHAs are biodegradable polymers with applications in packaging, coatings, and biomedical fields. The accumulation of P(HPE) can be induced by specific microbial species, showcasing the compound's potential in sustainable materials science .
Flavoring Agents
In the flavor and fragrance industry, this compound is recognized for its cheesy odor and dairy flavor profile. It is used as a flavoring agent in various food products, contributing to sensory experiences in culinary applications .
Chemical Intermediates
The compound plays a crucial role as an intermediate in the synthesis of various chemical products. Its reactivity allows it to participate in numerous reactions leading to valuable derivatives used across multiple industries, including pharmaceuticals and agrochemicals .
Case Study 1: Inhibition of Fatty Acid Oxidation
A study investigated the effects of this compound on mitochondrial fatty acid oxidation rates. Results indicated that higher concentrations of the compound significantly inhibited oxidation processes, suggesting potential applications in metabolic research and therapeutic development for conditions like obesity and diabetes .
Case Study 2: Scale-Up of Bromolactonization
Research focused on scaling up the bromolactonization reaction involving this compound demonstrated that optimizing substrate concentrations could enhance product yields while minimizing waste. The findings are critical for industrial applications where efficiency and sustainability are paramount .
Data Table: Summary of Applications
Application Area | Description | Key Findings/Insights |
---|---|---|
Biochemical Studies | Inhibition of fatty acid oxidation | Significant inhibition observed in mitochondrial studies |
Chemoenzymatic Reactions | Bromolactonization at preparative scale | Over 90% yield achieved with optimized conditions |
PHA Production | Precursor for biodegradable polymers | Induced by specific microbial strains |
Flavoring Agents | Used as a flavoring component with a cheesy odor | Enhances sensory profiles in food products |
Chemical Intermediates | Intermediate for various chemical syntheses | Valuable for pharmaceuticals and agrochemicals |
Mechanism of Action
The mechanism of action of 4-pentenoic acid involves its ability to inhibit fatty acid oxidation. This is achieved by its conversion to a CoA ester, which then inhibits the oxidation of long-chain fatty acids in mitochondria . This inhibition is primarily due to the depletion of CoA, which is essential for fatty acid metabolism .
Comparison with Similar Compounds
Comparison with Structurally and Functionally Similar Compounds
Methylenecyclopropylacetic Acid (MCPA)
- Structural Similarity : Both 4-PA and MCPA are unsaturated carboxylic acids. MCPA is a hypoglycin A metabolite linked to Jamaican vomiting sickness, while 4-PA mimics its toxic effects .
- Functional Differences: MCPA directly inhibits β-oxidation enzymes, whereas 4-PA’s toxicity arises from its metabolite, 2,4-pentadienoyl-CoA, which irreversibly inhibits mitochondrial thiolase .
Propionic Acid
- Structural Similarity : Both are short-chain carboxylic acids.
- Propionic acid’s hyperammonemia arises from CoA sequestration, while 4-PA disrupts mitochondrial energy metabolism and ATP synthesis .
Valproic Acid (VPA) and 4-ene VPA
- Structural Similarity : 4-PA is a structural analog of 4-ene VPA, a hepatotoxic metabolite of VPA .
- Functional Differences: 4-ene VPA forms glutathione conjugates (e.g., 3-oxo-4-ene VPA) linked to hepatotoxicity, while 4-PA generates 3-oxo-4-pentenoic acid conjugates . 4-PA induces mitochondrial permeability transition (MPT) at lower concentrations than VPA, contributing to its acute toxicity .
Fluorinated Analogs (e.g., α-Fluoro-4-ene VPA)
- Structural Similarity : Fluorination at the α-position blocks β-oxidation, making these analogs inert to mitochondrial degradation .
- Functional Differences :
Octanoic Acid
- Structural Similarity : Both are medium-chain fatty acids.
- 4-PA uniquely reduces mitochondrial ATP and ornithine transport, exacerbating hyperammonemia .
Key Research Findings and Data Tables
Table 1: Inhibitory Effects on Mitochondrial Pathways
Table 2: Toxicity Profiles in Animal Models
Compound | Hyperammonemia | Hypoglycemia | Microvesicular Steatosis | Mitochondrial MPT Induction |
---|---|---|---|---|
This compound | Yes | Yes | Yes | Yes (≥5 mM) |
Propionic acid | Yes | No | Yes | No |
4-ene VPA | Yes | Yes | Yes | Yes (≥10 mM) |
Octanoic acid | Mild | No | Yes | No |
Mechanistic Insights and Contradictions
- Enzyme Inhibition : 4-PA inhibits carbamyl phosphate synthetase (CPS) and OTC in rats only after preincubation, suggesting indirect effects via energy depletion . This contrasts with direct inhibition observed in human OTC .
- Conflicting Data : Some studies attribute 4-PA’s ureagenesis inhibition to ATP depletion, while others propose direct enzyme modulation .
Biological Activity
4-Pentenoic acid, an unsaturated fatty acid, has garnered attention in biochemical research due to its diverse biological activities. It is a structural isomer of pentanoic acid and has been studied for its metabolic effects, potential therapeutic applications, and interactions with various biological systems. This article compiles findings from multiple studies to provide a comprehensive overview of the biological activity of this compound.
- Chemical Formula : C₅H₸O₂
- Molecular Weight : 100.13 g/mol
- Structure : Contains a double bond between the fourth and fifth carbon atoms, contributing to its reactivity and biological effects.
Metabolic Effects
This compound has been shown to influence metabolic pathways significantly. Research indicates that it can inhibit gluconeogenesis and fatty acid oxidation in various cellular models. Notably, studies on Tetrahymena pyriformis demonstrated that this compound inhibited glucose utilization and altered lipid metabolism. The inhibition was consistent across different substrates, suggesting a broad impact on energy metabolism pathways .
Case Studies
- Gluconeogenesis Inhibition :
-
Cellular Toxicity :
- Research highlighted that this compound exhibits convulsant activity by acting as an inhibitor of glutamate decarboxylase (GAD), which is crucial for GABA synthesis. This suggests that high concentrations could lead to neurotoxic effects, emphasizing the need for careful dosage in therapeutic applications .
Enzymatic Interactions
This compound interacts with various enzymes, affecting their activity:
- Inhibition of Glycogen Synthesis : Similar to pentanoate, it inhibits glycogen synthesis from multiple substrates, impacting energy storage mechanisms in cells .
- Effect on Biocatalysts : In a study scaling up the bromolactonization of this compound, it was found that high concentrations led to significant enzyme inhibition, highlighting its potential as a substrate in enzymatic reactions but also its limitations due to substrate inhibition .
Industrial Uses
This compound is being explored for its applications in the production of polyhydroxyalkanoates (PHAs), biodegradable polymers synthesized by microbial fermentation. These materials are gaining traction due to their environmental benefits and versatility in applications ranging from packaging to biomedical devices .
Biomedical Applications
The compound's ability to modulate metabolic pathways positions it as a candidate for developing treatments for metabolic diseases. Its role in inhibiting gluconeogenesis may be particularly relevant for conditions like diabetes.
Summary of Findings
Study Focus | Key Findings |
---|---|
Metabolic Pathways | Inhibits gluconeogenesis and fatty acid oxidation; impacts energy metabolism significantly. |
Neurotoxicity | Acts as a GAD inhibitor; potential convulsant activity noted at high concentrations. |
Enzymatic Interactions | Significant substrate inhibition observed in biocatalytic processes; affects glycogen synthesis. |
Industrial Applications | Explored in PHA production; potential for biodegradable material synthesis. |
Q & A
Basic Research Questions
Q. What are the standard analytical techniques for quantifying 4-pentenoic acid in biological samples, and how can their accuracy be validated?
- Methodological Answer : High-performance liquid chromatography (HPLC) and gas chromatography-mass spectrometry (GC-MS) are widely used for quantification. Calibration curves using pure standards and spike-recovery experiments in matrices (e.g., plasma, urine) are critical for validation. For structural confirmation, tandem mass spectrometry (MS/MS) can differentiate this compound from structural analogs like valeric acid by analyzing fragmentation patterns (e.g., cyclic carbonium ions unique to this compound) .
Q. What storage conditions are recommended to preserve this compound’s stability in laboratory settings?
- Methodological Answer : Store at 2–8°C in airtight, light-resistant containers to prevent degradation. Solubility in alcohol (ethanol, methanol) allows preparation of stock solutions, but aqueous solutions should be prepared fresh due to hydrolysis risks. Monitor pH stability, as its pKa (4.677 at 25°C) influences ionization in aqueous environments .
Q. How can researchers synthesize this compound, and what purity assessment methods are recommended?
- Methodological Answer : Common synthesis routes include oxidation of 4-penten-1-ol or carboxylation of allylic intermediates. Purity is assessed via nuclear magnetic resonance (NMR) for structural verification and gas chromatography (GC) for quantification of residual solvents. Thermodynamic properties (e.g., boiling point: ~193°F) guide distillation-based purification .
Advanced Research Questions
Q. What experimental models are most effective for investigating this compound’s role in drug-induced hepatotoxicity?
- Methodological Answer : In vitro models (e.g., primary hepatocytes or HepG2 cells) treated with this compound can assess mitochondrial dysfunction via ATP assays. In vivo rodent models with co-administration of valproic acid (VPA) allow evaluation of hepatotoxicity biomarkers (e.g., glutathione depletion, ALT/AST levels). Use LC-MS to detect reactive metabolites like 4-ene-VPA conjugates with glutathione, which are implicated in toxicity .
Q. How does this compound’s terminal double bond influence its metabolic pathways, and what techniques can track these transformations?
- Methodological Answer : The double bond enables β-oxidation to 2,4-pentadienoyl-CoA, detectable via isotopic labeling and mass spectrometry. Stable isotope tracing (e.g., ¹³C-labeled this compound) in mitochondrial extracts can map intermediates. MS/MS is critical for identifying cyclic carbonium ions formed during intramolecular cyclization, a reaction absent in saturated analogs .
Q. What conflicting hypotheses exist about this compound’s mechanism of reducing renal ATP, and how can researchers resolve these discrepancies?
- Methodological Answer : Hypothesis 1: ATP depletion is mediated via adenosine accumulation from altered nucleotide metabolism. Hypothesis 2: Direct inhibition of mitochondrial complexes reduces ATP synthesis. To resolve this, use kidney perfusion models with adenosine receptor antagonists (e.g., theophylline) and measure ATP via luciferase assays. Compare results with mitochondrial respirometry data to isolate the primary mechanism .
Q. How can computational chemistry enhance the understanding of this compound’s thermodynamic and kinetic behavior in reactions?
- Methodological Answer : Density functional theory (DFT) calculations predict reaction pathways (e.g., cyclization energetics) using software like Gaussian. Validate predictions with experimental kinetics (e.g., Arrhenius plots for thermal decomposition) and compare with NIST-reported thermodynamic data (e.g., ΔvapH° = 44.8 kJ/mol) .
Q. Data Contradiction Analysis
- Discrepancy in Hepatotoxicity Mechanisms : While some studies attribute toxicity to glutathione depletion via 4-ene-VPA conjugates, others suggest direct mitochondrial inhibition. Researchers should design dual-pathway inhibition experiments (e.g., glutathione supplementation + mitochondrial complex inhibitors) to quantify relative contributions .
Properties
IUPAC Name |
pent-4-enoic acid | |
---|---|---|
Source | PubChem | |
URL | https://pubchem.ncbi.nlm.nih.gov | |
Description | Data deposited in or computed by PubChem | |
InChI |
InChI=1S/C5H8O2/c1-2-3-4-5(6)7/h2H,1,3-4H2,(H,6,7) | |
Source | PubChem | |
URL | https://pubchem.ncbi.nlm.nih.gov | |
Description | Data deposited in or computed by PubChem | |
InChI Key |
HVAMZGADVCBITI-UHFFFAOYSA-N | |
Source | PubChem | |
URL | https://pubchem.ncbi.nlm.nih.gov | |
Description | Data deposited in or computed by PubChem | |
Canonical SMILES |
C=CCCC(=O)O | |
Source | PubChem | |
URL | https://pubchem.ncbi.nlm.nih.gov | |
Description | Data deposited in or computed by PubChem | |
Molecular Formula |
C5H8O2 | |
Source | PubChem | |
URL | https://pubchem.ncbi.nlm.nih.gov | |
Description | Data deposited in or computed by PubChem | |
DSSTOX Substance ID |
DTXSID0044448 | |
Record name | 4-Pentenoic acid | |
Source | EPA DSSTox | |
URL | https://comptox.epa.gov/dashboard/DTXSID0044448 | |
Description | DSSTox provides a high quality public chemistry resource for supporting improved predictive toxicology. | |
Molecular Weight |
100.12 g/mol | |
Source | PubChem | |
URL | https://pubchem.ncbi.nlm.nih.gov | |
Description | Data deposited in or computed by PubChem | |
Physical Description |
Liquid, colourless liquid with a cheese-like odour | |
Record name | 4-Pentenoic acid | |
Source | Human Metabolome Database (HMDB) | |
URL | http://www.hmdb.ca/metabolites/HMDB0031602 | |
Description | The Human Metabolome Database (HMDB) is a freely available electronic database containing detailed information about small molecule metabolites found in the human body. | |
Explanation | HMDB is offered to the public as a freely available resource. Use and re-distribution of the data, in whole or in part, for commercial purposes requires explicit permission of the authors and explicit acknowledgment of the source material (HMDB) and the original publication (see the HMDB citing page). We ask that users who download significant portions of the database cite the HMDB paper in any resulting publications. | |
Record name | 4-Pentenoic acid | |
Source | Joint FAO/WHO Expert Committee on Food Additives (JECFA) | |
URL | https://www.fao.org/food/food-safety-quality/scientific-advice/jecfa/jecfa-flav/details/en/c/378/ | |
Description | The flavoring agent databse provides the most recent specifications for flavorings evaluated by JECFA. | |
Explanation | Permission from WHO is not required for the use of WHO materials issued under the Creative Commons Attribution-NonCommercial-ShareAlike 3.0 Intergovernmental Organization (CC BY-NC-SA 3.0 IGO) licence. | |
Solubility |
slightly soluble in water; soluble in alcohol and ether | |
Record name | 4-Pentenoic acid | |
Source | Joint FAO/WHO Expert Committee on Food Additives (JECFA) | |
URL | https://www.fao.org/food/food-safety-quality/scientific-advice/jecfa/jecfa-flav/details/en/c/378/ | |
Description | The flavoring agent databse provides the most recent specifications for flavorings evaluated by JECFA. | |
Explanation | Permission from WHO is not required for the use of WHO materials issued under the Creative Commons Attribution-NonCommercial-ShareAlike 3.0 Intergovernmental Organization (CC BY-NC-SA 3.0 IGO) licence. | |
Density |
0.970-0.990 (20°) | |
Record name | 4-Pentenoic acid | |
Source | Joint FAO/WHO Expert Committee on Food Additives (JECFA) | |
URL | https://www.fao.org/food/food-safety-quality/scientific-advice/jecfa/jecfa-flav/details/en/c/378/ | |
Description | The flavoring agent databse provides the most recent specifications for flavorings evaluated by JECFA. | |
Explanation | Permission from WHO is not required for the use of WHO materials issued under the Creative Commons Attribution-NonCommercial-ShareAlike 3.0 Intergovernmental Organization (CC BY-NC-SA 3.0 IGO) licence. | |
CAS No. |
591-80-0 | |
Record name | 4-Pentenoic acid | |
Source | CAS Common Chemistry | |
URL | https://commonchemistry.cas.org/detail?cas_rn=591-80-0 | |
Description | CAS Common Chemistry is an open community resource for accessing chemical information. Nearly 500,000 chemical substances from CAS REGISTRY cover areas of community interest, including common and frequently regulated chemicals, and those relevant to high school and undergraduate chemistry classes. This chemical information, curated by our expert scientists, is provided in alignment with our mission as a division of the American Chemical Society. | |
Explanation | The data from CAS Common Chemistry is provided under a CC-BY-NC 4.0 license, unless otherwise stated. | |
Record name | 4-Pentenoic acid | |
Source | ChemIDplus | |
URL | https://pubchem.ncbi.nlm.nih.gov/substance/?source=chemidplus&sourceid=0000591800 | |
Description | ChemIDplus is a free, web search system that provides access to the structure and nomenclature authority files used for the identification of chemical substances cited in National Library of Medicine (NLM) databases, including the TOXNET system. | |
Record name | Pent-4-enoic acid | |
Source | DTP/NCI | |
URL | https://dtp.cancer.gov/dtpstandard/servlet/dwindex?searchtype=NSC&outputformat=html&searchlist=20944 | |
Description | The NCI Development Therapeutics Program (DTP) provides services and resources to the academic and private-sector research communities worldwide to facilitate the discovery and development of new cancer therapeutic agents. | |
Explanation | Unless otherwise indicated, all text within NCI products is free of copyright and may be reused without our permission. Credit the National Cancer Institute as the source. | |
Record name | Pent-4-enoic acid | |
Source | DTP/NCI | |
URL | https://dtp.cancer.gov/dtpstandard/servlet/dwindex?searchtype=NSC&outputformat=html&searchlist=9000 | |
Description | The NCI Development Therapeutics Program (DTP) provides services and resources to the academic and private-sector research communities worldwide to facilitate the discovery and development of new cancer therapeutic agents. | |
Explanation | Unless otherwise indicated, all text within NCI products is free of copyright and may be reused without our permission. Credit the National Cancer Institute as the source. | |
Record name | 4-Pentenoic acid | |
Source | EPA Chemicals under the TSCA | |
URL | https://www.epa.gov/chemicals-under-tsca | |
Description | EPA Chemicals under the Toxic Substances Control Act (TSCA) collection contains information on chemicals and their regulations under TSCA, including non-confidential content from the TSCA Chemical Substance Inventory and Chemical Data Reporting. | |
Record name | 4-Pentenoic acid | |
Source | EPA DSSTox | |
URL | https://comptox.epa.gov/dashboard/DTXSID0044448 | |
Description | DSSTox provides a high quality public chemistry resource for supporting improved predictive toxicology. | |
Record name | Pent-4-enoic acid | |
Source | European Chemicals Agency (ECHA) | |
URL | https://echa.europa.eu/substance-information/-/substanceinfo/100.008.849 | |
Description | The European Chemicals Agency (ECHA) is an agency of the European Union which is the driving force among regulatory authorities in implementing the EU's groundbreaking chemicals legislation for the benefit of human health and the environment as well as for innovation and competitiveness. | |
Explanation | Use of the information, documents and data from the ECHA website is subject to the terms and conditions of this Legal Notice, and subject to other binding limitations provided for under applicable law, the information, documents and data made available on the ECHA website may be reproduced, distributed and/or used, totally or in part, for non-commercial purposes provided that ECHA is acknowledged as the source: "Source: European Chemicals Agency, http://echa.europa.eu/". Such acknowledgement must be included in each copy of the material. ECHA permits and encourages organisations and individuals to create links to the ECHA website under the following cumulative conditions: Links can only be made to webpages that provide a link to the Legal Notice page. | |
Record name | 4-PENTENOIC ACID | |
Source | FDA Global Substance Registration System (GSRS) | |
URL | https://gsrs.ncats.nih.gov/ginas/app/beta/substances/D4S77Y29FB | |
Description | The FDA Global Substance Registration System (GSRS) enables the efficient and accurate exchange of information on what substances are in regulated products. Instead of relying on names, which vary across regulatory domains, countries, and regions, the GSRS knowledge base makes it possible for substances to be defined by standardized, scientific descriptions. | |
Explanation | Unless otherwise noted, the contents of the FDA website (www.fda.gov), both text and graphics, are not copyrighted. They are in the public domain and may be republished, reprinted and otherwise used freely by anyone without the need to obtain permission from FDA. Credit to the U.S. Food and Drug Administration as the source is appreciated but not required. | |
Record name | 4-Pentenoic acid | |
Source | Human Metabolome Database (HMDB) | |
URL | http://www.hmdb.ca/metabolites/HMDB0031602 | |
Description | The Human Metabolome Database (HMDB) is a freely available electronic database containing detailed information about small molecule metabolites found in the human body. | |
Explanation | HMDB is offered to the public as a freely available resource. Use and re-distribution of the data, in whole or in part, for commercial purposes requires explicit permission of the authors and explicit acknowledgment of the source material (HMDB) and the original publication (see the HMDB citing page). We ask that users who download significant portions of the database cite the HMDB paper in any resulting publications. | |
Melting Point |
-22.5 °C | |
Record name | 4-Pentenoic acid | |
Source | Human Metabolome Database (HMDB) | |
URL | http://www.hmdb.ca/metabolites/HMDB0031602 | |
Description | The Human Metabolome Database (HMDB) is a freely available electronic database containing detailed information about small molecule metabolites found in the human body. | |
Explanation | HMDB is offered to the public as a freely available resource. Use and re-distribution of the data, in whole or in part, for commercial purposes requires explicit permission of the authors and explicit acknowledgment of the source material (HMDB) and the original publication (see the HMDB citing page). We ask that users who download significant portions of the database cite the HMDB paper in any resulting publications. | |
Synthesis routes and methods I
Procedure details
Synthesis routes and methods II
Procedure details
Synthesis routes and methods III
Procedure details
Retrosynthesis Analysis
AI-Powered Synthesis Planning: Our tool employs the Template_relevance Pistachio, Template_relevance Bkms_metabolic, Template_relevance Pistachio_ringbreaker, Template_relevance Reaxys, Template_relevance Reaxys_biocatalysis model, leveraging a vast database of chemical reactions to predict feasible synthetic routes.
One-Step Synthesis Focus: Specifically designed for one-step synthesis, it provides concise and direct routes for your target compounds, streamlining the synthesis process.
Accurate Predictions: Utilizing the extensive PISTACHIO, BKMS_METABOLIC, PISTACHIO_RINGBREAKER, REAXYS, REAXYS_BIOCATALYSIS database, our tool offers high-accuracy predictions, reflecting the latest in chemical research and data.
Strategy Settings
Precursor scoring | Relevance Heuristic |
---|---|
Min. plausibility | 0.01 |
Model | Template_relevance |
Template Set | Pistachio/Bkms_metabolic/Pistachio_ringbreaker/Reaxys/Reaxys_biocatalysis |
Top-N result to add to graph | 6 |
Feasible Synthetic Routes
Disclaimer and Information on In-Vitro Research Products
Please be aware that all articles and product information presented on BenchChem are intended solely for informational purposes. The products available for purchase on BenchChem are specifically designed for in-vitro studies, which are conducted outside of living organisms. In-vitro studies, derived from the Latin term "in glass," involve experiments performed in controlled laboratory settings using cells or tissues. It is important to note that these products are not categorized as medicines or drugs, and they have not received approval from the FDA for the prevention, treatment, or cure of any medical condition, ailment, or disease. We must emphasize that any form of bodily introduction of these products into humans or animals is strictly prohibited by law. It is essential to adhere to these guidelines to ensure compliance with legal and ethical standards in research and experimentation.