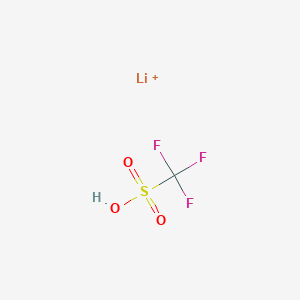
Lithium trifluoromethanesulfonate
- Click on QUICK INQUIRY to receive a quote from our team of experts.
- With the quality product at a COMPETITIVE price, you can focus more on your research.
Overview
Description
Lithium trifluoromethanesulfonate, also known as lithium triflate, is a chemical compound with the formula CF₃SO₃Li. It consists of a lithium cation (Li⁺) and a triflate anion (CF₃SO₃⁻). This compound is highly hygroscopic, meaning it readily absorbs moisture from the air. It is widely used in various applications, particularly in the field of lithium-ion batteries due to its excellent ionic conductivity .
Mechanism of Action
Target of Action
Lithium trifluoromethanesulfonate, also known as lithium triflate, is a salt composed of the lithium cation (Li+) and triflate anion (CF3SO3−; TfO−) . It is primarily used in the production of lithium-ion batteries .
Mode of Action
The lithium cation (Li+) in this compound interacts with the triflate anion (CF3SO3−; TfO−) to form a very hygroscopic salt . This hygroscopic nature allows the salt to absorb water from air humidity , which can influence its behavior in various applications, such as in lithium-ion batteries.
Pharmacokinetics
Given its use in lithium-ion batteries, its bioavailability is likely influenced by factors such as its hygroscopic nature and its stability under various environmental conditions .
Result of Action
In the context of lithium-ion batteries, the action of this compound enables the formation of greener and sustainable batteries for electrical energy storage . It improves the electrochemical and mechanical characteristics of the battery’s membrane .
Action Environment
Environmental factors can significantly influence the action, efficacy, and stability of this compound. For example, its hygroscopic nature means that it readily absorbs water from air humidity . This can affect its behavior in applications like lithium-ion batteries, where moisture can influence the efficiency and lifespan of the battery.
Biochemical Analysis
Biochemical Properties
It is known that the compound is highly soluble in water and organic solvents This solubility allows it to interact with various biomolecules in biochemical reactions
Cellular Effects
It is known that the compound plays a crucial role in lithium-ion battery production This suggests that it may influence cell function, potentially impacting cell signaling pathways, gene expression, and cellular metabolism
Molecular Mechanism
It is known that the compound is composed of the lithium cation (Li+) and triflate anion (CF3SO3−) . The triflate anion owes its stability to resonance stabilization, which causes the negative charge to be spread symmetrically over the three oxygen atoms . An additional stabilization is achieved by the trifluoromethyl group, which acts as a strong electron-withdrawing group using the sulfur atom as a bridge .
Temporal Effects in Laboratory Settings
It is known that the compound is very hygroscopic and absorbs water from air humidity This suggests that it may have certain stability and degradation characteristics over time
Metabolic Pathways
Given its role in lithium-ion battery production , it may interact with certain enzymes or cofactors and influence metabolic flux or metabolite levels
Transport and Distribution
Given its high solubility in water and organic solvents , it may interact with certain transporters or binding proteins and influence its localization or accumulation
Preparation Methods
Synthetic Routes and Reaction Conditions
Lithium trifluoromethanesulfonate can be synthesized through several methods. One common method involves the reaction of trifluoromethanesulfonic acid with lithium hydroxide or lithium carbonate. The reaction typically occurs in an aqueous medium and results in the formation of this compound and water as a byproduct .
Industrial Production Methods
In industrial settings, this compound is often produced by reacting liquid sulfur trioxide with trifluoromethane gas in an inert gas atmosphere. This method ensures high purity and yield of the product .
Chemical Reactions Analysis
Types of Reactions
Lithium trifluoromethanesulfonate undergoes various chemical reactions, including:
Substitution Reactions: It can act as a source of the triflate anion in substitution reactions, where it replaces other anions in a compound.
Catalytic Reactions: It serves as a catalyst in several organic reactions, such as the Friedlander synthesis of quinolines.
Common Reagents and Conditions
Common reagents used in reactions involving this compound include organic solvents like acetonitrile and dichloromethane. The reactions are typically carried out under anhydrous conditions to prevent the compound from absorbing moisture .
Major Products Formed
The major products formed from reactions involving this compound depend on the specific reaction. For example, in the Friedlander synthesis, the major products are quinoline derivatives .
Scientific Research Applications
Lithium trifluoromethanesulfonate has a wide range of scientific research applications:
Comparison with Similar Compounds
Similar Compounds
- Lithium tetrafluoroborate (LiBF₄)
- Lithium hexafluorophosphate (LiPF₆)
- Lithium bis(trifluoromethanesulfonyl)imide (LiTFSI)
Uniqueness
Lithium trifluoromethanesulfonate is unique due to its high thermal stability and excellent ionic conductivity. Unlike lithium tetrafluoroborate and lithium hexafluorophosphate, it is less prone to hydrolysis, making it more suitable for applications in humid environments . Additionally, its triflate anion provides superior stability compared to other anions, enhancing its performance in various applications .
Biological Activity
Lithium trifluoromethanesulfonate (LiTf), also known as lithium triflate, is a lithium salt with the formula LiCF₃SO₃. It has garnered attention in various fields due to its unique chemical properties and potential biological activities. This article explores the biological activity of LiTf, focusing on its applications in biopolymer systems, electrochemical properties, and potential therapeutic uses.
- Molecular Formula : CF₃LiO₃S
- Molecular Weight : 156.01 g/mol
- Density : 1.9 g/cm³
- Melting Point : >300 °C
- Solubility : Soluble in water and various organic solvents
LiTf is characterized by its high hygroscopicity and stability, making it suitable for various applications, including as an electrolyte in lithium-ion batteries and in organic synthesis as a catalyst .
1. Biopolymer Electrolytes
Recent studies have investigated the incorporation of LiTf into biopolymer matrices, such as cassava starch and pectin, to enhance their ionic conductivity and structural properties. The following table summarizes key findings from relevant research:
The addition of LiTf alters the interaction between polymer chains, reducing hydrogen bonding and promoting flexibility, which is crucial for applications in solid polymer electrolytes (SPEs).
2. Electrochemical Behavior
LiTf has been shown to influence the electrochemical properties of biopolymer films significantly. For example, the presence of LiTf affects the redox potentials of glucose units in biopolymers, enhancing their ionic conductivity and making them suitable for energy storage applications .
3. Catalytic Activity
In addition to its role in biopolymers, LiTf serves as an efficient catalyst for various organic reactions. It has been used successfully in the acetylation of alcohols and diacetylation of aldehydes under mild conditions, yielding good to excellent results without affecting sensitive functional groups . This catalytic property may have implications for drug synthesis and development.
Case Study 1: Lithium Triflate in Bone Healing
A study evaluated the effects of this compound on bone healing properties using a rabbit model. The results indicated that treatment with LiTf enhanced osteogenic potential compared to control groups. However, no significant differences were observed between treated groups regarding biomechanical strength .
Case Study 2: Gamma Irradiation Effects
Research using electron spin resonance (ESR) spectroscopy investigated the effects of gamma radiation on LiTf. The findings revealed changes in the radical species formed upon irradiation, suggesting potential applications for LiTf in radioprotective strategies or in understanding radiation-induced biological effects .
Properties
CAS No. |
33454-82-9 |
---|---|
Molecular Formula |
CHF3LiO3S |
Molecular Weight |
157.0 g/mol |
IUPAC Name |
lithium;trifluoromethanesulfonate |
InChI |
InChI=1S/CHF3O3S.Li/c2-1(3,4)8(5,6)7;/h(H,5,6,7); |
InChI Key |
WDGKXRCNMKPDSD-UHFFFAOYSA-N |
SMILES |
[Li+].C(F)(F)(F)S(=O)(=O)[O-] |
Isomeric SMILES |
[Li+].C(F)(F)(F)S(=O)(=O)[O-] |
Canonical SMILES |
[Li].C(F)(F)(F)S(=O)(=O)O |
Key on ui other cas no. |
33454-82-9 |
Pictograms |
Irritant |
Related CAS |
1493-13-6 (Parent) |
Synonyms |
AgOTf cpd Al(OTf)3 aluminum triflate As(otf)2 cerium triflate Cu(OTf)2 cupric trifluoromethanesulfonate Eu(OTf)3 In(OTf)3 indium triflate mercury(II) trifluoromethanesulfonate samarium triflate silver triflate silver trifluoromethanesulfonate TFMSA cpd triflic acid trifluoromethanesulfonate trifluoromethanesulfonic acid trifluoromethanesulfonic acid, aluminum salt trifluoromethanesulfonic acid, barium salt trifluoromethanesulfonic acid, cerium (+3) salt trifluoromethanesulfonic acid, cupric salt trifluoromethanesulfonic acid, indium salt trifluoromethanesulfonic acid, lanthanum (+3) salt trifluoromethanesulfonic acid, lithium salt trifluoromethanesulfonic acid, samarium salt trifluoromethanesulfonic acid, silver (+1) salt trifluoromethanesulfonic acid, zinc salt zinc triflate |
Origin of Product |
United States |
Retrosynthesis Analysis
AI-Powered Synthesis Planning: Our tool employs the Template_relevance Pistachio, Template_relevance Bkms_metabolic, Template_relevance Pistachio_ringbreaker, Template_relevance Reaxys, Template_relevance Reaxys_biocatalysis model, leveraging a vast database of chemical reactions to predict feasible synthetic routes.
One-Step Synthesis Focus: Specifically designed for one-step synthesis, it provides concise and direct routes for your target compounds, streamlining the synthesis process.
Accurate Predictions: Utilizing the extensive PISTACHIO, BKMS_METABOLIC, PISTACHIO_RINGBREAKER, REAXYS, REAXYS_BIOCATALYSIS database, our tool offers high-accuracy predictions, reflecting the latest in chemical research and data.
Strategy Settings
Precursor scoring | Relevance Heuristic |
---|---|
Min. plausibility | 0.01 |
Model | Template_relevance |
Template Set | Pistachio/Bkms_metabolic/Pistachio_ringbreaker/Reaxys/Reaxys_biocatalysis |
Top-N result to add to graph | 6 |
Feasible Synthetic Routes
Disclaimer and Information on In-Vitro Research Products
Please be aware that all articles and product information presented on BenchChem are intended solely for informational purposes. The products available for purchase on BenchChem are specifically designed for in-vitro studies, which are conducted outside of living organisms. In-vitro studies, derived from the Latin term "in glass," involve experiments performed in controlled laboratory settings using cells or tissues. It is important to note that these products are not categorized as medicines or drugs, and they have not received approval from the FDA for the prevention, treatment, or cure of any medical condition, ailment, or disease. We must emphasize that any form of bodily introduction of these products into humans or animals is strictly prohibited by law. It is essential to adhere to these guidelines to ensure compliance with legal and ethical standards in research and experimentation.