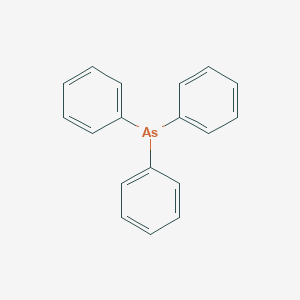
Triphenylarsine
Overview
Description
Triphenylarsine is an organoarsenic compound with the chemical formula As(C₆H₅)₃. It is a colorless crystalline solid that is primarily used as a ligand and reagent in coordination chemistry and organic synthesis . The molecule has a pyramidal structure with arsenic-carbon distances ranging from 1.942 to 1.956 Å and carbon-arsenic-carbon angles between 99.6 and 100.5 degrees .
Mechanism of Action
Target of Action
Triphenylarsine is an organoarsenic compound that is primarily used as a ligand and a reagent in coordination chemistry and organic synthesis . It forms metal complexes with metals, most of which are analogues of the corresponding triphenylphosphine derivatives .
Mode of Action
This compound is known to accelerate the reaction rate of the Stille coupling reaction . This reaction is a key process in organic synthesis, where it is used to form carbon-carbon bonds between two organic groups . The this compound ligand interacts with its targets, facilitating the coupling reaction and leading to the formation of the desired product .
Biochemical Pathways
It is known that the compound plays a crucial role in the stille coupling reaction . This reaction is a part of larger synthetic pathways used in the production of various organic compounds .
Result of Action
The primary result of this compound’s action is the acceleration of the Stille coupling reaction . This leads to the efficient formation of carbon-carbon bonds, facilitating the synthesis of various organic compounds .
Action Environment
The action, efficacy, and stability of this compound can be influenced by various environmental factors. These include the temperature and pressure under which the reactions are carried out, the presence of other compounds, and the specific conditions of the reaction environment .
Biochemical Analysis
Biochemical Properties
Triphenylarsine is known to be used as a ligand in coordination chemistry and organic synthesis
Cellular Effects
It is known that organoarsenic compounds can have various effects on cells, including influencing cell function, cell signaling pathways, gene expression, and cellular metabolism
Molecular Mechanism
It is known to form metal complexes with metals, most of which are analogues of the corresponding triphenylphosphine derivatives
Preparation Methods
Synthetic Routes and Reaction Conditions: Triphenylarsine is typically synthesized by the reaction of arsenic trichloride with chlorobenzene in the presence of sodium as a reducing agent . The reaction can be represented as follows:
AsCl3+3PhCl+6Na→AsPh3+6NaCl
Industrial Production Methods: In industrial settings, the synthesis of this compound follows similar routes but on a larger scale. The reaction conditions are optimized to ensure high yield and purity of the product. The use of sodium as a reducing agent is common due to its effectiveness in facilitating the reaction.
Types of Reactions:
Oxidation: this compound can undergo oxidation to form this compound oxide.
Reduction: It can be reduced to form lithium diphenylarsenide when reacted with lithium.
Substitution: this compound can participate in substitution reactions to form various organoarsenic compounds.
Common Reagents and Conditions:
Oxidation: Common oxidizing agents include bromine and hydrogen peroxide.
Reduction: Lithium is a common reducing agent used in the formation of lithium diphenylarsenide.
Substitution: Reagents such as bromine and organomagnesium compounds are often used.
Major Products:
Oxidation: this compound oxide.
Reduction: Lithium diphenylarsenide.
Substitution: Various organoarsenic compounds, including tetraphenylarsonium chloride.
Scientific Research Applications
Triphenylarsine finds applications in various fields of scientific research:
Chemistry: It is used as a ligand in the synthesis of metal complexes and as a reagent in organic synthesis.
Biology: this compound derivatives are studied for their potential biological activities.
Medicine: Research is ongoing to explore the medicinal properties of organoarsenic compounds.
Industry: It is used in the synthesis of polymers and as a catalyst in various chemical reactions.
Comparison with Similar Compounds
Triphenylphosphine: Similar in structure but contains phosphorus instead of arsenic.
Triphenylstibine: Contains antimony instead of arsenic.
Triphenylamine: Contains nitrogen instead of arsenic.
Triphenylborane: Contains boron instead of arsenic.
Uniqueness: Triphenylarsine is unique due to the presence of arsenic, which imparts distinct chemical properties compared to its phosphorus, antimony, nitrogen, and boron analogs. The arsenic atom in this compound has a higher inversion barrier than nitrogen, making it more stable and easier to handle in air compared to other organoarsenic compounds .
Properties
IUPAC Name |
triphenylarsane | |
---|---|---|
Source | PubChem | |
URL | https://pubchem.ncbi.nlm.nih.gov | |
Description | Data deposited in or computed by PubChem | |
InChI |
InChI=1S/C18H15As/c1-4-10-16(11-5-1)19(17-12-6-2-7-13-17)18-14-8-3-9-15-18/h1-15H | |
Source | PubChem | |
URL | https://pubchem.ncbi.nlm.nih.gov | |
Description | Data deposited in or computed by PubChem | |
InChI Key |
BPLUKJNHPBNVQL-UHFFFAOYSA-N | |
Source | PubChem | |
URL | https://pubchem.ncbi.nlm.nih.gov | |
Description | Data deposited in or computed by PubChem | |
Canonical SMILES |
C1=CC=C(C=C1)[As](C2=CC=CC=C2)C3=CC=CC=C3 | |
Source | PubChem | |
URL | https://pubchem.ncbi.nlm.nih.gov | |
Description | Data deposited in or computed by PubChem | |
Molecular Formula |
C18H15As | |
Source | PubChem | |
URL | https://pubchem.ncbi.nlm.nih.gov | |
Description | Data deposited in or computed by PubChem | |
DSSTOX Substance ID |
DTXSID4060529 | |
Record name | Arsine, triphenyl- | |
Source | EPA DSSTox | |
URL | https://comptox.epa.gov/dashboard/DTXSID4060529 | |
Description | DSSTox provides a high quality public chemistry resource for supporting improved predictive toxicology. | |
Molecular Weight |
306.2 g/mol | |
Source | PubChem | |
URL | https://pubchem.ncbi.nlm.nih.gov | |
Description | Data deposited in or computed by PubChem | |
Physical Description |
White powder; [Alfa Aesar MSDS] Fine colorless crystals; [Aldrich MSDS] | |
Record name | Triphenylarsine | |
Source | Haz-Map, Information on Hazardous Chemicals and Occupational Diseases | |
URL | https://haz-map.com/Agents/9544 | |
Description | Haz-Map® is an occupational health database designed for health and safety professionals and for consumers seeking information about the adverse effects of workplace exposures to chemical and biological agents. | |
Explanation | Copyright (c) 2022 Haz-Map(R). All rights reserved. Unless otherwise indicated, all materials from Haz-Map are copyrighted by Haz-Map(R). No part of these materials, either text or image may be used for any purpose other than for personal use. Therefore, reproduction, modification, storage in a retrieval system or retransmission, in any form or by any means, electronic, mechanical or otherwise, for reasons other than personal use, is strictly prohibited without prior written permission. | |
Vapor Pressure |
0.0000496 [mmHg] | |
Record name | Triphenylarsine | |
Source | Haz-Map, Information on Hazardous Chemicals and Occupational Diseases | |
URL | https://haz-map.com/Agents/9544 | |
Description | Haz-Map® is an occupational health database designed for health and safety professionals and for consumers seeking information about the adverse effects of workplace exposures to chemical and biological agents. | |
Explanation | Copyright (c) 2022 Haz-Map(R). All rights reserved. Unless otherwise indicated, all materials from Haz-Map are copyrighted by Haz-Map(R). No part of these materials, either text or image may be used for any purpose other than for personal use. Therefore, reproduction, modification, storage in a retrieval system or retransmission, in any form or by any means, electronic, mechanical or otherwise, for reasons other than personal use, is strictly prohibited without prior written permission. | |
CAS No. |
603-32-7 | |
Record name | Triphenylarsine | |
Source | CAS Common Chemistry | |
URL | https://commonchemistry.cas.org/detail?cas_rn=603-32-7 | |
Description | CAS Common Chemistry is an open community resource for accessing chemical information. Nearly 500,000 chemical substances from CAS REGISTRY cover areas of community interest, including common and frequently regulated chemicals, and those relevant to high school and undergraduate chemistry classes. This chemical information, curated by our expert scientists, is provided in alignment with our mission as a division of the American Chemical Society. | |
Explanation | The data from CAS Common Chemistry is provided under a CC-BY-NC 4.0 license, unless otherwise stated. | |
Record name | Triphenylarsine | |
Source | ChemIDplus | |
URL | https://pubchem.ncbi.nlm.nih.gov/substance/?source=chemidplus&sourceid=0000603327 | |
Description | ChemIDplus is a free, web search system that provides access to the structure and nomenclature authority files used for the identification of chemical substances cited in National Library of Medicine (NLM) databases, including the TOXNET system. | |
Record name | TRIPHENYLARSINE | |
Source | DTP/NCI | |
URL | https://dtp.cancer.gov/dtpstandard/servlet/dwindex?searchtype=NSC&outputformat=html&searchlist=478 | |
Description | The NCI Development Therapeutics Program (DTP) provides services and resources to the academic and private-sector research communities worldwide to facilitate the discovery and development of new cancer therapeutic agents. | |
Explanation | Unless otherwise indicated, all text within NCI products is free of copyright and may be reused without our permission. Credit the National Cancer Institute as the source. | |
Record name | Arsine, triphenyl- | |
Source | EPA Chemicals under the TSCA | |
URL | https://www.epa.gov/chemicals-under-tsca | |
Description | EPA Chemicals under the Toxic Substances Control Act (TSCA) collection contains information on chemicals and their regulations under TSCA, including non-confidential content from the TSCA Chemical Substance Inventory and Chemical Data Reporting. | |
Record name | Arsine, triphenyl- | |
Source | EPA DSSTox | |
URL | https://comptox.epa.gov/dashboard/DTXSID4060529 | |
Description | DSSTox provides a high quality public chemistry resource for supporting improved predictive toxicology. | |
Record name | Triphenylarsine | |
Source | European Chemicals Agency (ECHA) | |
URL | https://echa.europa.eu/substance-information/-/substanceinfo/100.009.121 | |
Description | The European Chemicals Agency (ECHA) is an agency of the European Union which is the driving force among regulatory authorities in implementing the EU's groundbreaking chemicals legislation for the benefit of human health and the environment as well as for innovation and competitiveness. | |
Explanation | Use of the information, documents and data from the ECHA website is subject to the terms and conditions of this Legal Notice, and subject to other binding limitations provided for under applicable law, the information, documents and data made available on the ECHA website may be reproduced, distributed and/or used, totally or in part, for non-commercial purposes provided that ECHA is acknowledged as the source: "Source: European Chemicals Agency, http://echa.europa.eu/". Such acknowledgement must be included in each copy of the material. ECHA permits and encourages organisations and individuals to create links to the ECHA website under the following cumulative conditions: Links can only be made to webpages that provide a link to the Legal Notice page. | |
Record name | TRIPHENYLARSINE | |
Source | FDA Global Substance Registration System (GSRS) | |
URL | https://gsrs.ncats.nih.gov/ginas/app/beta/substances/MN8EZ3FL74 | |
Description | The FDA Global Substance Registration System (GSRS) enables the efficient and accurate exchange of information on what substances are in regulated products. Instead of relying on names, which vary across regulatory domains, countries, and regions, the GSRS knowledge base makes it possible for substances to be defined by standardized, scientific descriptions. | |
Explanation | Unless otherwise noted, the contents of the FDA website (www.fda.gov), both text and graphics, are not copyrighted. They are in the public domain and may be republished, reprinted and otherwise used freely by anyone without the need to obtain permission from FDA. Credit to the U.S. Food and Drug Administration as the source is appreciated but not required. | |
Synthesis routes and methods
Procedure details
Retrosynthesis Analysis
AI-Powered Synthesis Planning: Our tool employs the Template_relevance Pistachio, Template_relevance Bkms_metabolic, Template_relevance Pistachio_ringbreaker, Template_relevance Reaxys, Template_relevance Reaxys_biocatalysis model, leveraging a vast database of chemical reactions to predict feasible synthetic routes.
One-Step Synthesis Focus: Specifically designed for one-step synthesis, it provides concise and direct routes for your target compounds, streamlining the synthesis process.
Accurate Predictions: Utilizing the extensive PISTACHIO, BKMS_METABOLIC, PISTACHIO_RINGBREAKER, REAXYS, REAXYS_BIOCATALYSIS database, our tool offers high-accuracy predictions, reflecting the latest in chemical research and data.
Strategy Settings
Precursor scoring | Relevance Heuristic |
---|---|
Min. plausibility | 0.01 |
Model | Template_relevance |
Template Set | Pistachio/Bkms_metabolic/Pistachio_ringbreaker/Reaxys/Reaxys_biocatalysis |
Top-N result to add to graph | 6 |
Feasible Synthetic Routes
Q1: What is the molecular formula and weight of Triphenylarsine?
A1: this compound has the molecular formula C18H15As and a molecular weight of 306.24 g/mol.
Q2: What spectroscopic data is available for this compound?
A2: Several spectroscopic techniques have been employed to characterize this compound. These include:
- NMR spectroscopy: Both 1H and 13C NMR spectra provide valuable information about the structure and bonding within the molecule. [, ]
- Infrared (IR) spectroscopy: IR spectra reveal characteristic vibrational frequencies associated with specific functional groups within this compound. [, , ]
- Mass spectrometry: Mass spectrometry studies provide insights into the fragmentation pattern of this compound under electron bombardment, helping to elucidate its structure. []
- UV-Vis Spectroscopy: UV-Vis spectra can reveal information about electronic transitions within the molecule, including potential conjugation effects. []
Q3: What solvents are commonly used with this compound?
A3: this compound exhibits solubility in various organic solvents, including:
- Toluene: Used for reactions with sulphuryl chloride to form this compound dichloride. []
- Dichloromethane: Employed in reactions with platinum complexes and diphenacyl sulphoxide. []
- Acetonitrile: Utilized in reactions with iron compounds and dioxygen for the synthesis of this compound oxide. [, ]
- Chloroform: A common solvent for extraction studies involving this compound and its derivatives. [, ]
Q4: Can this compound act as a catalyst?
A5: Yes, this compound has been shown to exhibit catalytic activity in certain organic reactions. For instance, it effectively catalyzes the cyclopropanation of alkenes with phenacyl bromide to produce trans-2,3-dihydro-spiro[cyclopropane-1,2′-indan-1′,3′-dione] with high stereoselectivity. []
Q5: How does this compound compare to Triphenylphosphine in catalytic applications?
A6: this compound and triphenylphosphine share some similarities in their coordination chemistry but can display different reactivity. For example, in palladium-catalyzed Suzuki cross-coupling reactions, polymer-supported this compound reagents were found to be effective ligands, simplifying product purification and enabling ligand recycling. [] While both can act as ligands, differences in steric and electronic properties can influence their catalytic activity and selectivity in various reactions.
Q6: Have computational methods been used to study this compound?
A6: Yes, computational chemistry has played a role in understanding this compound and related compounds. For example:
- Bond strength calculations: G3 calculations have been used to estimate the As=S bond strength in this compound sulfide, providing insights into its reactivity and potential for sulfur atom transfer reactions. []
- Molecular orbital calculations: PM3(tm) calculations have been employed to investigate the electronic structure of (hydrotris(pyrazolyl)borato)(this compound)copper(I). These calculations helped to assign the lowest energy electronic transition as a ligand-to-ligand charge transfer (LLCT) process. []
Q7: How do structural modifications of this compound affect its properties?
A7: While the provided research doesn't delve deeply into systematic SAR studies, some observations highlight the impact of structural modifications:
- Oxidation to this compound oxide: This modification significantly alters its coordination chemistry. This compound oxide readily forms complexes with various metal ions, such as tin, lead, and iron. [, , , , ]
- Substitution on the phenyl rings: Studies on the extraction of zinc(II) and europium(III) using thenoyltrifluoroacetone and this compound oxide derivatives revealed that the electron-donating ability of the arsine oxide ligand, influenced by substituents on the phenyl rings, significantly impacts the extraction efficiency. []
Q8: Are there any specific formulation strategies mentioned for this compound or its derivatives?
A8: While the provided research primarily focuses on fundamental chemistry and doesn't extensively cover formulation aspects, it's worth noting that:
- Polymer-supported this compound reagents: These have been developed and showcase improved practicality for Suzuki cross-coupling reactions. The polymeric support simplifies product purification and allows for ligand recycling. [] This highlights a strategy to enhance the utility of this compound derivatives in synthetic applications.
Q9: What are the SHE considerations for this compound?
A9: As with all chemicals, handling this compound requires adherence to safety guidelines. Specific safety data sheets should always be consulted. Key points include:
Q10: What types of complexes does this compound form?
A10: this compound acts as a ligand in various metal complexes, including:
- Halogen-bridged dimers: Examples include [(Ph3As)2Cu(μ-X)2Cu(AsPh3)2].2CHCl3, where X = Cl or Br. [] These complexes often exhibit interesting structural features and have been characterized by X-ray crystallography.
- Oxo-bridged diiron(III) complexes: The reaction of this compound with dioxygen in the presence of iron compounds and bromide ions leads to the formation of [(OAsPh3)4Fe(μ-O)FeBr3]+ [FeBr4]- ·CH3CN. This complex exhibits an unsymmetrical Fe-O-Fe bridge and has been characterized structurally. []
- Complexes with other ligands: this compound can coordinate to metal centers alongside other ligands, such as triphenylphosphine and crown thioethers, forming mixed-ligand complexes. [, ]
Q11: Does this compound react with oxygen?
A12: Yes, this compound can be oxidized to this compound oxide, especially in the presence of catalysts like iron compounds. [, ] This oxidation reaction is often highly selective and can be performed under mild conditions.
Q12: How does the basicity of this compound oxide compare to other ligands?
A13: this compound oxide demonstrates stronger electron-donating properties compared to its phosphorus analogue, Triphenylphosphine oxide. This difference in Lewis basicity influences its ability to form adducts with metal complexes and impacts extraction efficiencies in solvent extraction processes. []
Q13: Are there any historical milestones related to this compound research?
A14: While the provided research doesn't delve into a historical overview, the early work by Kaoru Matsumiya and Minoru Nakaz, published in 1926, explored the reaction of this compound with arsenic trisulphide. [] This research provided foundational knowledge about the reactivity of this compound and its ability to form various arsenic-containing compounds.
Disclaimer and Information on In-Vitro Research Products
Please be aware that all articles and product information presented on BenchChem are intended solely for informational purposes. The products available for purchase on BenchChem are specifically designed for in-vitro studies, which are conducted outside of living organisms. In-vitro studies, derived from the Latin term "in glass," involve experiments performed in controlled laboratory settings using cells or tissues. It is important to note that these products are not categorized as medicines or drugs, and they have not received approval from the FDA for the prevention, treatment, or cure of any medical condition, ailment, or disease. We must emphasize that any form of bodily introduction of these products into humans or animals is strictly prohibited by law. It is essential to adhere to these guidelines to ensure compliance with legal and ethical standards in research and experimentation.