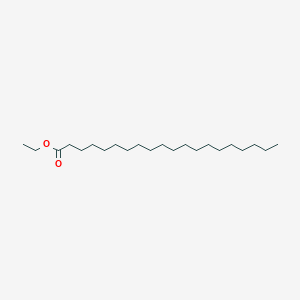
Ethyl arachidate
Overview
Description
Ethyl arachidate is a long-chain fatty acid ethyl ester resulting from the formal condensation of the carboxy group of arachidic (icosanoic) acid with the hydroxy group of ethanol . It is functionally related to an icosanoic acid .
Synthesis Analysis
The synthesis of Ethyl arachidate involves the formal condensation of the carboxy group of arachidic (icosanoic) acid with the hydroxy group of ethanol .
Molecular Structure Analysis
The molecular formula of Ethyl arachidate is C22H44O2 . It is a long-chain fatty acid ethyl ester . The molecular weight of Ethyl arachidate is 340.6 g/mol .
Physical And Chemical Properties Analysis
Ethyl arachidate is a solid at 20 degrees Celsius . It has a melting point range of 41.0 to 45.0 degrees Celsius . The exact mass and monoisotopic mass of Ethyl arachidate are 340.334130642 g/mol . It has a XLogP3-AA value of 10, indicating its lipophilicity .
Scientific Research Applications
Flavoring and Fragrance Agent
Ethyl arachidate is used as a flavoring agent due to its fruity, waxy smell. It’s commonly added to foods like baked goods, candy, and beverages to enhance their taste. Additionally, it serves as a fragrance ingredient in personal care products .
Lubricant and Plasticizer
In industrial applications, Ethyl arachidate functions as a lubricant or plasticizer. Its chemical properties make it suitable for use in machinery and manufacturing processes where smooth operation is critical .
Synthesis of Organic Compounds
As a starting material, Ethyl arachidate is involved in the synthesis of other organic compounds. Its structure allows for various chemical reactions, making it a valuable resource in organic chemistry research .
Biochemical Research Reagent
In life science research, Ethyl arachidate is utilized as a biochemical reagent. It can be used as a biological material or organic compound for experiments and studies related to cellular and molecular biology .
Anti-Inflammatory and Analgesic Research
Ethyl arachidate has been studied for its anti-inflammatory and analgesic effects. Research suggests that it can inhibit inflammation and reduce pain, which justifies its use in traditional medicine and opens up possibilities for pharmaceutical applications .
Material Science
Scientists in material science explore the use of Ethyl arachidate in the development of new materials. Its properties could contribute to creating materials with specific desired characteristics, such as enhanced durability or flexibility .
Mechanism of Action
Ethyl arachidate, also known as Ethyl icosanoate, is a long-chain fatty acid ethyl ester . This compound is a product of the formal condensation of the carboxy group of arachidic (icosanoic) acid with the hydroxy group of ethanol .
Target of Action
The compound is a long-chain fatty acid ethyl ester, which suggests that it may interact with various enzymes and receptors in the body that are involved in lipid metabolism .
Mode of Action
As a long-chain fatty acid ethyl ester, it is likely to be involved in various biological processes, including signal transduction, contraction, chemotaxis, and cell proliferation, differentiation, and apoptosis .
Pharmacokinetics
As a lipid compound, it is likely to be absorbed in the intestines, distributed in the body through the bloodstream, metabolized in the liver, and excreted through the kidneys .
Result of Action
It is known that long-chain fatty acid ethyl esters like ethyl arachidate can have various effects on cells, including influencing membrane fluidity and function, modulating enzyme activity, and affecting gene expression .
Action Environment
The action, efficacy, and stability of Ethyl arachidate can be influenced by various environmental factors. For example, the presence of other lipids and proteins can affect its absorption and distribution in the body. Additionally, factors such as pH and temperature can influence its stability .
properties
IUPAC Name |
ethyl icosanoate | |
---|---|---|
Source | PubChem | |
URL | https://pubchem.ncbi.nlm.nih.gov | |
Description | Data deposited in or computed by PubChem | |
InChI |
InChI=1S/C22H44O2/c1-3-5-6-7-8-9-10-11-12-13-14-15-16-17-18-19-20-21-22(23)24-4-2/h3-21H2,1-2H3 | |
Source | PubChem | |
URL | https://pubchem.ncbi.nlm.nih.gov | |
Description | Data deposited in or computed by PubChem | |
InChI Key |
YBKSMWBLSBAFBQ-UHFFFAOYSA-N | |
Source | PubChem | |
URL | https://pubchem.ncbi.nlm.nih.gov | |
Description | Data deposited in or computed by PubChem | |
Canonical SMILES |
CCCCCCCCCCCCCCCCCCCC(=O)OCC | |
Source | PubChem | |
URL | https://pubchem.ncbi.nlm.nih.gov | |
Description | Data deposited in or computed by PubChem | |
Molecular Formula |
C22H44O2 | |
Source | PubChem | |
URL | https://pubchem.ncbi.nlm.nih.gov | |
Description | Data deposited in or computed by PubChem | |
DSSTOX Substance ID |
DTXSID50171335 | |
Record name | Ethyl arachidate | |
Source | EPA DSSTox | |
URL | https://comptox.epa.gov/dashboard/DTXSID50171335 | |
Description | DSSTox provides a high quality public chemistry resource for supporting improved predictive toxicology. | |
Molecular Weight |
340.6 g/mol | |
Source | PubChem | |
URL | https://pubchem.ncbi.nlm.nih.gov | |
Description | Data deposited in or computed by PubChem | |
Product Name |
Ethyl arachidate | |
CAS RN |
18281-05-5 | |
Record name | Ethyl eicosanoate | |
Source | CAS Common Chemistry | |
URL | https://commonchemistry.cas.org/detail?cas_rn=18281-05-5 | |
Description | CAS Common Chemistry is an open community resource for accessing chemical information. Nearly 500,000 chemical substances from CAS REGISTRY cover areas of community interest, including common and frequently regulated chemicals, and those relevant to high school and undergraduate chemistry classes. This chemical information, curated by our expert scientists, is provided in alignment with our mission as a division of the American Chemical Society. | |
Explanation | The data from CAS Common Chemistry is provided under a CC-BY-NC 4.0 license, unless otherwise stated. | |
Record name | Ethyl arachidate | |
Source | ChemIDplus | |
URL | https://pubchem.ncbi.nlm.nih.gov/substance/?source=chemidplus&sourceid=0018281055 | |
Description | ChemIDplus is a free, web search system that provides access to the structure and nomenclature authority files used for the identification of chemical substances cited in National Library of Medicine (NLM) databases, including the TOXNET system. | |
Record name | Ethyl arachidate | |
Source | EPA DSSTox | |
URL | https://comptox.epa.gov/dashboard/DTXSID50171335 | |
Description | DSSTox provides a high quality public chemistry resource for supporting improved predictive toxicology. | |
Record name | ETHYL ARACHIDATE | |
Source | FDA Global Substance Registration System (GSRS) | |
URL | https://gsrs.ncats.nih.gov/ginas/app/beta/substances/KKI20ZX76X | |
Description | The FDA Global Substance Registration System (GSRS) enables the efficient and accurate exchange of information on what substances are in regulated products. Instead of relying on names, which vary across regulatory domains, countries, and regions, the GSRS knowledge base makes it possible for substances to be defined by standardized, scientific descriptions. | |
Explanation | Unless otherwise noted, the contents of the FDA website (www.fda.gov), both text and graphics, are not copyrighted. They are in the public domain and may be republished, reprinted and otherwise used freely by anyone without the need to obtain permission from FDA. Credit to the U.S. Food and Drug Administration as the source is appreciated but not required. | |
Retrosynthesis Analysis
AI-Powered Synthesis Planning: Our tool employs the Template_relevance Pistachio, Template_relevance Bkms_metabolic, Template_relevance Pistachio_ringbreaker, Template_relevance Reaxys, Template_relevance Reaxys_biocatalysis model, leveraging a vast database of chemical reactions to predict feasible synthetic routes.
One-Step Synthesis Focus: Specifically designed for one-step synthesis, it provides concise and direct routes for your target compounds, streamlining the synthesis process.
Accurate Predictions: Utilizing the extensive PISTACHIO, BKMS_METABOLIC, PISTACHIO_RINGBREAKER, REAXYS, REAXYS_BIOCATALYSIS database, our tool offers high-accuracy predictions, reflecting the latest in chemical research and data.
Strategy Settings
Precursor scoring | Relevance Heuristic |
---|---|
Min. plausibility | 0.01 |
Model | Template_relevance |
Template Set | Pistachio/Bkms_metabolic/Pistachio_ringbreaker/Reaxys/Reaxys_biocatalysis |
Top-N result to add to graph | 6 |
Feasible Synthetic Routes
Q & A
Q1: What is the significance of Ethyl arachidate being identified in Mucuna flagellipes seeds?
A1: The identification of Ethyl arachidate in Mucuna flagellipes seeds by GC-MS analysis contributes to the understanding of the phytochemical profile of this plant. [] This is particularly interesting because M. flagellipes is used in traditional medicine in Nigeria. The presence of Ethyl arachidate, along with other esters and carboxylic acids, may contribute to the aroma enhancement properties of the seeds when used in soups. [] Further research is needed to determine the specific biological activities and potential applications of Ethyl arachidate and other identified compounds in M. flagellipes.
Q2: Are there any analytical challenges in studying Ethyl arachidate?
A3: Ethyl arachidate, being a long-chain fatty acid ester, can be challenging to isolate and characterize from complex mixtures. [] In the study on Buddleja cordata subsp. cordata, researchers utilized a combination of bioassay-guided fractionation, HPLC, and GC-MS analysis to identify and characterize Ethyl arachidate alongside other similar esters. [] This highlights the need for sophisticated analytical techniques to accurately identify and quantify these compounds in various matrices.
Disclaimer and Information on In-Vitro Research Products
Please be aware that all articles and product information presented on BenchChem are intended solely for informational purposes. The products available for purchase on BenchChem are specifically designed for in-vitro studies, which are conducted outside of living organisms. In-vitro studies, derived from the Latin term "in glass," involve experiments performed in controlled laboratory settings using cells or tissues. It is important to note that these products are not categorized as medicines or drugs, and they have not received approval from the FDA for the prevention, treatment, or cure of any medical condition, ailment, or disease. We must emphasize that any form of bodily introduction of these products into humans or animals is strictly prohibited by law. It is essential to adhere to these guidelines to ensure compliance with legal and ethical standards in research and experimentation.