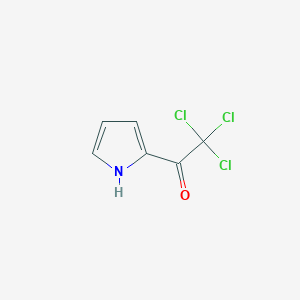
2-(Trichloroacetyl)pyrrole
Overview
Description
Mechanism of Action
Target of Action
2-(Trichloroacetyl)pyrrole is a 2-substituted pyrrole
Mode of Action
It’s known that the compound undergoes acylation to afford 4-acyl derivatives . This suggests that it might interact with its targets through acylation, leading to changes in the target’s function.
Biochemical Pathways
Given that it undergoes acylation to afford 4-acyl derivatives , it may influence pathways involving acylation reactions.
Result of Action
The acylation process it undergoes could potentially lead to modifications in target molecules, influencing their function .
Preparation Methods
Synthetic Routes and Reaction Conditions: 2-(Trichloroacetyl)pyrrole can be synthesized through the acylation of pyrrole with trichloroacetyl chloride. The reaction typically involves the use of a base such as pyridine to neutralize the hydrochloric acid formed during the reaction .
Industrial Production Methods: In an industrial setting, the synthesis of this compound may involve continuous flow processes to ensure consistent quality and yield. The reaction conditions are optimized to maintain the purity of the product, often requiring precise temperature control and the use of high-purity reagents .
Chemical Reactions Analysis
Types of Reactions: 2-(Trichloroacetyl)pyrrole undergoes various types of chemical reactions, including:
Acylation: It can be acylated to form 4-acyl derivatives, such as 4-formyl to 4-hexanoyl.
Substitution: The trichloroacetyl group can be substituted with other functional groups under appropriate conditions.
Common Reagents and Conditions:
Acylation: Reagents such as acyl chlorides and bases like pyridine are commonly used.
Substitution: Nucleophiles such as amines or alcohols can be used to replace the trichloroacetyl group.
Major Products:
Acylation Products: 4-acyl derivatives of pyrrole.
Substitution Products: Various substituted pyrrole derivatives depending on the nucleophile used.
Scientific Research Applications
2-(Trichloroacetyl)pyrrole is utilized in several scientific research applications, including:
Comparison with Similar Compounds
2-Acetylpyrrole: Similar in structure but with an acetyl group instead of a trichloroacetyl group.
2-Propionylpyrrole: Contains a propionyl group instead of a trichloroacetyl group.
2-(Trifluoroacetyl)pyrrole: Contains a trifluoroacetyl group, offering different reactivity and properties.
Uniqueness: 2-(Trichloroacetyl)pyrrole is unique due to its trichloroacetyl group, which imparts distinct reactivity and allows for the formation of specific acyl derivatives that are not easily accessible with other acyl groups .
Properties
IUPAC Name |
2,2,2-trichloro-1-(1H-pyrrol-2-yl)ethanone | |
---|---|---|
Source | PubChem | |
URL | https://pubchem.ncbi.nlm.nih.gov | |
Description | Data deposited in or computed by PubChem | |
InChI |
InChI=1S/C6H4Cl3NO/c7-6(8,9)5(11)4-2-1-3-10-4/h1-3,10H | |
Source | PubChem | |
URL | https://pubchem.ncbi.nlm.nih.gov | |
Description | Data deposited in or computed by PubChem | |
InChI Key |
BBFDGMDENAEMKF-UHFFFAOYSA-N | |
Source | PubChem | |
URL | https://pubchem.ncbi.nlm.nih.gov | |
Description | Data deposited in or computed by PubChem | |
Canonical SMILES |
C1=CNC(=C1)C(=O)C(Cl)(Cl)Cl | |
Source | PubChem | |
URL | https://pubchem.ncbi.nlm.nih.gov | |
Description | Data deposited in or computed by PubChem | |
Molecular Formula |
C6H4Cl3NO | |
Source | PubChem | |
URL | https://pubchem.ncbi.nlm.nih.gov | |
Description | Data deposited in or computed by PubChem | |
DSSTOX Substance ID |
DTXSID70313578 | |
Record name | 2-(Trichloroacetyl)pyrrole | |
Source | EPA DSSTox | |
URL | https://comptox.epa.gov/dashboard/DTXSID70313578 | |
Description | DSSTox provides a high quality public chemistry resource for supporting improved predictive toxicology. | |
Molecular Weight |
212.5 g/mol | |
Source | PubChem | |
URL | https://pubchem.ncbi.nlm.nih.gov | |
Description | Data deposited in or computed by PubChem | |
CAS No. |
35302-72-8 | |
Record name | 35302-72-8 | |
Source | DTP/NCI | |
URL | https://dtp.cancer.gov/dtpstandard/servlet/dwindex?searchtype=NSC&outputformat=html&searchlist=272669 | |
Description | The NCI Development Therapeutics Program (DTP) provides services and resources to the academic and private-sector research communities worldwide to facilitate the discovery and development of new cancer therapeutic agents. | |
Explanation | Unless otherwise indicated, all text within NCI products is free of copyright and may be reused without our permission. Credit the National Cancer Institute as the source. | |
Record name | 2-(Trichloroacetyl)pyrrole | |
Source | EPA DSSTox | |
URL | https://comptox.epa.gov/dashboard/DTXSID70313578 | |
Description | DSSTox provides a high quality public chemistry resource for supporting improved predictive toxicology. | |
Record name | 2-(Trichloroacetyl)pyrrole | |
Source | European Chemicals Agency (ECHA) | |
URL | https://echa.europa.eu/information-on-chemicals | |
Description | The European Chemicals Agency (ECHA) is an agency of the European Union which is the driving force among regulatory authorities in implementing the EU's groundbreaking chemicals legislation for the benefit of human health and the environment as well as for innovation and competitiveness. | |
Explanation | Use of the information, documents and data from the ECHA website is subject to the terms and conditions of this Legal Notice, and subject to other binding limitations provided for under applicable law, the information, documents and data made available on the ECHA website may be reproduced, distributed and/or used, totally or in part, for non-commercial purposes provided that ECHA is acknowledged as the source: "Source: European Chemicals Agency, http://echa.europa.eu/". Such acknowledgement must be included in each copy of the material. ECHA permits and encourages organisations and individuals to create links to the ECHA website under the following cumulative conditions: Links can only be made to webpages that provide a link to the Legal Notice page. | |
Synthesis routes and methods I
Procedure details
Synthesis routes and methods II
Procedure details
Synthesis routes and methods III
Procedure details
Retrosynthesis Analysis
AI-Powered Synthesis Planning: Our tool employs the Template_relevance Pistachio, Template_relevance Bkms_metabolic, Template_relevance Pistachio_ringbreaker, Template_relevance Reaxys, Template_relevance Reaxys_biocatalysis model, leveraging a vast database of chemical reactions to predict feasible synthetic routes.
One-Step Synthesis Focus: Specifically designed for one-step synthesis, it provides concise and direct routes for your target compounds, streamlining the synthesis process.
Accurate Predictions: Utilizing the extensive PISTACHIO, BKMS_METABOLIC, PISTACHIO_RINGBREAKER, REAXYS, REAXYS_BIOCATALYSIS database, our tool offers high-accuracy predictions, reflecting the latest in chemical research and data.
Strategy Settings
Precursor scoring | Relevance Heuristic |
---|---|
Min. plausibility | 0.01 |
Model | Template_relevance |
Template Set | Pistachio/Bkms_metabolic/Pistachio_ringbreaker/Reaxys/Reaxys_biocatalysis |
Top-N result to add to graph | 6 |
Feasible Synthetic Routes
Q1: What is the primary use of 2-(Trichloroacetyl)pyrrole in scientific research?
A1: this compound serves as a versatile intermediate in synthesizing various compounds, particularly 2,4-disubstituted pyrroles. [] This is due to the reactivity of the trichloroacetyl group, which can be easily modified to introduce different substituents onto the pyrrole ring.
Q2: How does the structure of this compound lend itself to studying biological systems?
A2: this compound forms dimers through N-H•••O=C hydrogen bonding, a type of interaction crucial for protein stability. [] Studying the dimerization of this compound provides insights into the factors influencing protein structure and stability.
Q3: What spectroscopic techniques are commonly employed to characterize this compound?
A3: FTIR spectroscopy is frequently used to investigate the hydrogen bonding interactions of this compound, particularly in dimer formation. [] Additionally, NMR, IR, and MS analyses are used to characterize the structure of this compound and its derivatives. [, , ]
Q4: Has the interaction of this compound with metal surfaces been studied?
A4: Yes, studies have investigated the adsorption of this compound onto silver and gold surfaces using Surface-Enhanced Raman Scattering (SERS). [] These studies revealed that this compound chemisorbs onto these surfaces, suggesting potential applications in biosensor development.
Q5: Are there any crystallographic studies on this compound derivatives?
A5: Yes, several crystal structures of this compound derivatives have been determined using X-ray diffraction. These studies provide valuable insights into the molecular geometry, intermolecular interactions, and packing arrangements of these compounds. [, , , , ]
Q6: What is the significance of studying the hetero-association of this compound?
A6: Research has explored the hetero-association of this compound with toluene, determining the complexation constant and thermodynamic parameters (enthalpy and entropy) of the interaction. [] This information helps understand the compound's interaction with different solvents and its potential applications in separation processes.
Q7: Have any computational studies been performed on this compound?
A7: DFT (Density Functional Theory) calculations have been employed to study the interaction of this compound with silver and gold clusters. [] These calculations validate experimental findings regarding the adsorption of the compound on metal surfaces and provide insights into the nature of the interaction.
Q8: What are some examples of this compound derivatives synthesized and characterized in the literature?
A8: Several derivatives, including N-(1-Butylpyrrole-2-carbonyl)-amino acid methyl esters, 3-(4-Bromo-1H-pyrrole-2-carboxamido)propanoic acid, and Ethyl (4-bromo-1H-pyrrole-2-carboxamido)acetate, have been synthesized and characterized. [, , ] These derivatives highlight the versatility of this compound as a building block for synthesizing structurally diverse compounds.
Disclaimer and Information on In-Vitro Research Products
Please be aware that all articles and product information presented on BenchChem are intended solely for informational purposes. The products available for purchase on BenchChem are specifically designed for in-vitro studies, which are conducted outside of living organisms. In-vitro studies, derived from the Latin term "in glass," involve experiments performed in controlled laboratory settings using cells or tissues. It is important to note that these products are not categorized as medicines or drugs, and they have not received approval from the FDA for the prevention, treatment, or cure of any medical condition, ailment, or disease. We must emphasize that any form of bodily introduction of these products into humans or animals is strictly prohibited by law. It is essential to adhere to these guidelines to ensure compliance with legal and ethical standards in research and experimentation.