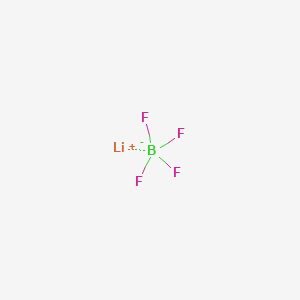
Lithium tetrafluoroborate
Overview
Description
Lithium tetrafluoroborate is an inorganic compound with the chemical formula LiBF₄. It appears as a white crystalline powder and is known for its high solubility in nonpolar solvents. This compound has been extensively tested for use in commercial secondary batteries, particularly lithium-ion batteries, due to its favorable properties such as high thermal stability and moisture tolerance .
Synthetic Routes and Reaction Conditions:
Industrial Production Methods:
Method 1: Industrially, this compound is produced as a byproduct in the synthesis of diborane.
Method 2: Another industrial method involves making hydrofluoric acid react with boric acid, followed by the addition of lithium carbonate or lithium hydroxide to obtain a this compound solution.
Types of Reactions:
Oxidation and Reduction: this compound can undergo oxidation and reduction reactions, particularly in the context of its use in lithium-ion batteries.
Substitution Reactions: It can act as a mild Lewis acid and participate in substitution reactions such as the Diels-Alder reaction and aminolysis of oxiranes.
Common Reagents and Conditions:
Reagents: Common reagents include lithium fluoride, boron trifluoride, hydrofluoric acid, boric acid, lithium carbonate, and lithium hydroxide
Conditions: Reactions typically occur under controlled temperatures and inert atmospheres to prevent unwanted side reactions and degradation.
Major Products:
From Oxidation and Reduction: The major products depend on the specific redox reactions involved in the battery applications.
From Substitution Reactions: Products vary based on the specific substitution reactions, such as the formation of new carbon-carbon bonds in Diels-Alder reactions.
Mechanism of Action
Target of Action
Lithium tetrafluoroborate (LiBF4) is primarily used as an electrolyte material in lithium-ion batteries . It targets the electrochemical processes within these batteries, specifically the ion transport mechanism .
Mode of Action
LiBF4 interacts with its target by providing a source of lithium ions, which are essential for the operation of lithium-ion batteries . The compound dissociates into lithium ions (Li+) and tetrafluoroborate ions (BF4-) in the electrolyte solution . The lithium ions then move from the anode to the cathode during discharge and vice versa during charging .
Biochemical Pathways
The movement of lithium ions from the anode to the cathode during discharge, and back during charging, is a fundamental process in the operation of lithium-ion batteries .
Pharmacokinetics
The term pharmacokinetics typically applies to the absorption, distribution, metabolism, and excretion (ADME) of drugs within a biological system. LiBF4 exhibits high solubility in nonpolar solvents, which aids in its distribution within the battery . Its reactions within the battery contribute to the overall electrochemical processes, and its stability under various conditions affects the battery’s performance .
Result of Action
The action of LiBF4 in a lithium-ion battery results in the generation of an electric current. This is achieved through the movement of lithium ions between the anode and cathode, facilitated by the LiBF4 in the electrolyte solution . The result at the molecular level is the oxidation and reduction reactions occurring at the anode and cathode, respectively .
Action Environment
The action of LiBF4 is influenced by various environmental factors. For instance, the presence of trace amounts of water in LiBF4 can cause its hydrolysis, leading to the generation of HF and the destruction of the battery electrodes . Additionally, LiBF4 exhibits greater thermal stability and moisture tolerance compared to other salts used in lithium-ion batteries, such as LiPF6 . This means that LiBF4 can tolerate a moisture content up to 620 ppm at room temperature .
Scientific Research Applications
Lithium tetrafluoroborate has several scientific research applications:
Chemistry: It is used as an electrolyte material in lithium-ion batteries, enhancing their performance and stability.
Biology and Medicine: While its direct applications in biology and medicine are limited, its role in battery technology indirectly supports medical devices that rely on lithium-ion batteries.
Comparison with Similar Compounds
- Lithium hexafluorophosphate (LiPF₆)
- Lithium difluoro(oxalato)borate (LiODFB)
- Lithium bis(oxalato)borate (LiBOB)
Comparison:
- Thermal Stability: Lithium tetrafluoroborate exhibits greater thermal stability compared to lithium hexafluorophosphate, which readily hydrolyzes into toxic gases .
- Moisture Tolerance: this compound can tolerate higher moisture content without degrading, unlike lithium hexafluorophosphate .
- Conductivity: While this compound has lower conductivity compared to some other lithium salts, its stability and safety make it a preferred choice in certain applications .
Properties
IUPAC Name |
lithium;tetrafluoroborate | |
---|---|---|
Source | PubChem | |
URL | https://pubchem.ncbi.nlm.nih.gov | |
Description | Data deposited in or computed by PubChem | |
InChI |
InChI=1S/BF4.Li/c2-1(3,4)5;/q-1;+1 | |
Source | PubChem | |
URL | https://pubchem.ncbi.nlm.nih.gov | |
Description | Data deposited in or computed by PubChem | |
InChI Key |
UFXJWFBILHTTET-UHFFFAOYSA-N | |
Source | PubChem | |
URL | https://pubchem.ncbi.nlm.nih.gov | |
Description | Data deposited in or computed by PubChem | |
Canonical SMILES |
[Li+].[B-](F)(F)(F)F | |
Source | PubChem | |
URL | https://pubchem.ncbi.nlm.nih.gov | |
Description | Data deposited in or computed by PubChem | |
Molecular Formula |
BF4Li | |
Source | PubChem | |
URL | https://pubchem.ncbi.nlm.nih.gov | |
Description | Data deposited in or computed by PubChem | |
Molecular Weight |
93.8 g/mol | |
Source | PubChem | |
URL | https://pubchem.ncbi.nlm.nih.gov | |
Description | Data deposited in or computed by PubChem | |
Physical Description |
White hygroscopic solid; [Merck Index] Off-white odorless powder; [Alfa Aesar MSDS] | |
Record name | Lithium tetrafluoroborate | |
Source | Haz-Map, Information on Hazardous Chemicals and Occupational Diseases | |
URL | https://haz-map.com/Agents/14759 | |
Description | Haz-Map® is an occupational health database designed for health and safety professionals and for consumers seeking information about the adverse effects of workplace exposures to chemical and biological agents. | |
Explanation | Copyright (c) 2022 Haz-Map(R). All rights reserved. Unless otherwise indicated, all materials from Haz-Map are copyrighted by Haz-Map(R). No part of these materials, either text or image may be used for any purpose other than for personal use. Therefore, reproduction, modification, storage in a retrieval system or retransmission, in any form or by any means, electronic, mechanical or otherwise, for reasons other than personal use, is strictly prohibited without prior written permission. | |
CAS No. |
14283-07-9 | |
Record name | Lithium tetrafluoroborate | |
Source | CAS Common Chemistry | |
URL | https://commonchemistry.cas.org/detail?cas_rn=14283-07-9 | |
Description | CAS Common Chemistry is an open community resource for accessing chemical information. Nearly 500,000 chemical substances from CAS REGISTRY cover areas of community interest, including common and frequently regulated chemicals, and those relevant to high school and undergraduate chemistry classes. This chemical information, curated by our expert scientists, is provided in alignment with our mission as a division of the American Chemical Society. | |
Explanation | The data from CAS Common Chemistry is provided under a CC-BY-NC 4.0 license, unless otherwise stated. | |
Record name | Borate(1-), tetrafluoro-, lithium (1:1) | |
Source | EPA Chemicals under the TSCA | |
URL | https://www.epa.gov/chemicals-under-tsca | |
Description | EPA Chemicals under the Toxic Substances Control Act (TSCA) collection contains information on chemicals and their regulations under TSCA, including non-confidential content from the TSCA Chemical Substance Inventory and Chemical Data Reporting. | |
Record name | Lithium tetrafluoroborate, anhydrous | |
Source | European Chemicals Agency (ECHA) | |
URL | https://echa.europa.eu/substance-information/-/substanceinfo/100.034.692 | |
Description | The European Chemicals Agency (ECHA) is an agency of the European Union which is the driving force among regulatory authorities in implementing the EU's groundbreaking chemicals legislation for the benefit of human health and the environment as well as for innovation and competitiveness. | |
Explanation | Use of the information, documents and data from the ECHA website is subject to the terms and conditions of this Legal Notice, and subject to other binding limitations provided for under applicable law, the information, documents and data made available on the ECHA website may be reproduced, distributed and/or used, totally or in part, for non-commercial purposes provided that ECHA is acknowledged as the source: "Source: European Chemicals Agency, http://echa.europa.eu/". Such acknowledgement must be included in each copy of the material. ECHA permits and encourages organisations and individuals to create links to the ECHA website under the following cumulative conditions: Links can only be made to webpages that provide a link to the Legal Notice page. | |
Record name | LITHIUM TETRAFLUOROBORATE | |
Source | FDA Global Substance Registration System (GSRS) | |
URL | https://gsrs.ncats.nih.gov/ginas/app/beta/substances/AF751CNK2N | |
Description | The FDA Global Substance Registration System (GSRS) enables the efficient and accurate exchange of information on what substances are in regulated products. Instead of relying on names, which vary across regulatory domains, countries, and regions, the GSRS knowledge base makes it possible for substances to be defined by standardized, scientific descriptions. | |
Explanation | Unless otherwise noted, the contents of the FDA website (www.fda.gov), both text and graphics, are not copyrighted. They are in the public domain and may be republished, reprinted and otherwise used freely by anyone without the need to obtain permission from FDA. Credit to the U.S. Food and Drug Administration as the source is appreciated but not required. | |
Q1: What are the advantages of using lithium tetrafluoroborate (LiBF4) as an electrolyte salt in lithium-ion batteries?
A1: LiBF4 exhibits several advantageous properties that make it suitable for lithium-ion batteries. It demonstrates good high-temperature storage performance and low-temperature discharging performance, thereby expanding the battery's operational temperature range []. Additionally, LiBF4 improves the charging upper limit voltage and high-temperature intermittent cycling performance of the battery, while simultaneously reducing the expansion rate and internal resistance, enhancing overall stability and safety [].
Q2: Are there any disadvantages of using LiBF4 as an electrolyte salt compared to other lithium salts?
A2: While LiBF4 offers advantages, it also has limitations compared to other lithium salts like lithium hexafluorophosphate (LiPF6) and Lithium bis(trifluoromethylsulfonyl)imide (LiTFSI). LiPF6-based electrolytes generally exhibit higher ionic conductivity than LiBF4-based electrolytes, especially at room temperature. LiTFSI offers better thermal stability and electrochemical stability at high voltages compared to LiBF4. The choice of the optimal lithium salt depends on the specific requirements of the battery application.
Q3: What is the effect of incorporating aluminum oxide (Al2O3) into a (PEO)6LiBF4 composite polymer electrolyte?
A3: Adding Al2O3 to (PEO)6LiBF4 composite polymer electrolyte significantly enhances ionic conductivity, up to ten times higher than without Al2O3. This improvement is observed across a temperature range of 30°C to 80°C. The inclusion of Al2O3 also considerably improves the stability of resistance between the electrode and electrolyte over time [].
Q4: What role does fumed silica play in composite poly(ethylene oxide) (PEO)-based polymer gel electrolytes containing LiBF4?
A4: In PEO-based polymer gel electrolytes, fumed silica influences the diffusion of ions. The presence of fumed silica can enhance the overall ionic conductivity of the electrolyte [].
Q5: Can this compound act as a catalyst in organic synthesis?
A5: Yes, this compound has proven to be an efficient catalyst in various organic reactions. It facilitates the conversion of aldehydes into geminal dicarboxylates (acylals) with good to excellent yields [].
Q6: What are some other reactions where this compound demonstrates catalytic activity?
A6: LiBF4 efficiently catalyzes the tetrahydropyranylation of alcohols [], promotes the synthesis of distillable 1,3-dithianes from aldehydes and ketones under solvent-free conditions [], and facilitates the formation of acetals from aldehydes and ketones with trialkyl orthoformates and the corresponding alcohol [].
Q7: What is the molecular formula and weight of this compound?
A7: this compound has the molecular formula LiBF4 and a molecular weight of 93.74 g/mol.
Q8: Are there any notable spectroscopic characteristics of this compound?
A8: Vibrational spectroscopic studies, specifically infrared and Raman spectroscopy, have been used to investigate the solvation and association interactions of LiBF4 in various solvents []. These studies provide insights into the behavior of LiBF4 in solution.
Q9: Have there been any computational studies on this compound and its interactions?
A9: Yes, molecular dynamics simulations have been employed to investigate the behavior of LiBF4 in various systems. These include studying the ion solvation and ion association of LiBF4 in 4-ethoxymethyl-ethylene carbonate [], the ionic liquid-borophene interface in the presence of LiBF4 [], and the structure of the graphene-ionic liquid/alkali salt mixtures interface with LiBF4 [].
Q10: What insights have these computational studies provided?
A10: Molecular dynamics simulations have revealed crucial information about the solvation behavior of LiBF4, its interactions with different materials like borophene and graphene, and the formation of ionic aggregates at various concentrations. These findings contribute to a deeper understanding of the fundamental properties and behavior of LiBF4 in different environments.
Q11: What are some alternatives to this compound in specific applications?
A11: Alternatives to LiBF4 are often dictated by the specific application:
- Lithium-ion batteries: Lithium hexafluorophosphate (LiPF6) remains a common electrolyte salt due to its high conductivity. Lithium bis(trifluoromethylsulfonyl)imide (LiTFSI) is gaining traction due to its wider electrochemical window and thermal stability. Lithium bis(oxalate)borate (LiBOB) presents itself as a safer alternative with good high-temperature performance [, ].
- Organic Synthesis: Depending on the specific reaction, other Lewis acid catalysts like boron trifluoride etherate, titanium tetrachloride, or aluminum chloride can be considered [].
Disclaimer and Information on In-Vitro Research Products
Please be aware that all articles and product information presented on BenchChem are intended solely for informational purposes. The products available for purchase on BenchChem are specifically designed for in-vitro studies, which are conducted outside of living organisms. In-vitro studies, derived from the Latin term "in glass," involve experiments performed in controlled laboratory settings using cells or tissues. It is important to note that these products are not categorized as medicines or drugs, and they have not received approval from the FDA for the prevention, treatment, or cure of any medical condition, ailment, or disease. We must emphasize that any form of bodily introduction of these products into humans or animals is strictly prohibited by law. It is essential to adhere to these guidelines to ensure compliance with legal and ethical standards in research and experimentation.