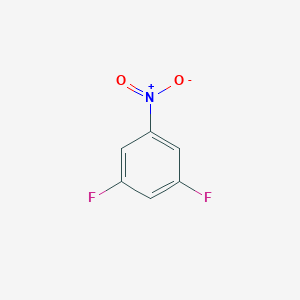
3,5-Difluoronitrobenzene
Overview
Description
3,5-Difluoronitrobenzene (CAS: 2265-94-3) is a fluorinated nitroaromatic compound with the molecular formula C₆H₃F₂NO₂ and a molecular weight of 159.09 g/mol . It is a pale-yellow liquid with a purity typically exceeding 99% . The compound features two fluorine atoms at the 3- and 5-positions and a nitro group at the 1-position of the benzene ring. Its structure has been extensively characterized using gas-phase electron diffraction (GED) and quantum chemical calculations, which reveal distinct bond lengths and angles influenced by fluorine's electron-withdrawing effects .
This compound serves as a key intermediate in pharmaceuticals and agrochemicals. For example, it is used in the synthesis of hydrazoarenes (85% yield via sodium borohydride reduction) and in coupling reactions to generate derivatives like 3,5-difluoro-N-(4-methoxyphenyl)aniline (79% yield) .
Preparation Methods
Halogen Exchange Reactions
Starting Materials and Reagents
Halogen exchange reactions are pivotal for introducing fluorine atoms into aromatic systems. A prominent route begins with 2,4,5-trichloronitrobenzene , which undergoes sequential fluorination and dechlorination. Alkali metal fluorides—such as potassium fluoride (KF) or cesium fluoride (CsF)—are employed in polar aprotic solvents like dimethyl sulfoxide (DMSO) or dimethylformamide (DMF) . These solvents enhance fluoride ion nucleophilicity, facilitating chlorine-to-fluorine substitution.
For example, reacting 2,4,5-trichloronitrobenzene with KF in DMF at 150°C for 12 hours yields 5-chloro-2,4-difluoronitrobenzene as an intermediate . The selectivity for fluorine substitution at the 2- and 4-positions is attributed to the electronic effects of the nitro group, which directs incoming fluoride ions to meta and para positions relative to itself.
Reaction Conditions and Catalysts
Phase-transfer catalysts (PTCs), such as tetrabutylammonium bromide, are occasionally added to improve reaction kinetics . Elevated temperatures (100–200°C) and prolonged reaction times (12–24 hours) are typical, ensuring complete substitution. The use of CsF instead of KF can reduce reaction times by 30% due to its higher solubility in aprotic media .
Table 1: Halogen Exchange Conditions for Fluorinated Nitrobenzene Synthesis
Nitration of Fluorinated Aromatic Compounds
Nitrating Agents and Conditions
Nitration introduces the nitro group into pre-fluorinated aromatic systems. A mixed acid system—comprising concentrated sulfuric acid (H₂SO₄) and nitric acid (HNO₃)—is standard. The sulfuric acid protonates the aromatic ring, enhancing electrophilic attack by the nitronium ion (NO₂⁺) .
In one protocol, 1,3-dichloro-4,6-difluorobenzene is nitrated at 30–40°C using a 2:1 (v/v) H₂SO₄/HNO₃ mixture. The reaction is exothermic, requiring careful temperature control to prevent polynitration or decomposition. After 4 hours, the crude product is diluted with ice water, and the organic layer is extracted with toluene .
Selectivity and Isomer Formation
Nitration regioselectivity depends on the directing effects of existing substituents. For 1,3-dichloro-4,6-difluorobenzene , the nitro group preferentially occupies the position para to chlorine atoms, yielding 2,6-dichloro-3,5-difluoronitrobenzene . Isomer separation is achieved via fractional distillation or column chromatography, though industrial processes often proceed without purification to minimize costs.
Catalytic Dechlorination
Catalyst Systems
Catalytic dechlorination removes residual chlorine atoms from intermediates like 2,6-dichloro-3,5-difluoronitrobenzene . Palladium-based catalysts, particularly 5% Pd/C (palladium on activated charcoal), are effective under hydrogen gas (H₂) . The reaction proceeds via oxidative addition of C–Cl bonds to palladium, followed by reductive elimination of HCl.
A typical procedure involves stirring 2,6-dichloro-3,5-difluoronitrobenzene with 5% Pd/C in ethanol at 80°C under 3 bar H₂ pressure for 6 hours. The catalyst is filtered, and the product is isolated by vacuum distillation .
Optimization of Reaction Parameters
Key factors influencing dechlorination efficiency include:
-
Catalyst loading : 0.5–5 wt% Pd/C relative to substrate.
-
Hydrogen pressure : 1–5 bar; higher pressures accelerate reaction rates.
-
Solvent selection : Ethanol or methanol improves catalyst dispersion and H₂ solubility.
Table 2: Dechlorination of 2,6-Dichloro-3,5-difluoronitrobenzene
Catalyst | Solvent | H₂ Pressure (bar) | Temperature (°C) | Time (h) | Yield (%) |
---|---|---|---|---|---|
5% Pd/C | Ethanol | 3 | 80 | 6 | 92 |
3% Pd/BaSO₄ | Methanol | 2 | 70 | 8 | 85 |
Comparative Analysis of Synthetic Routes
Yield Comparisons
-
Halogen exchange + nitration : Overall yields of 65–78% due to multi-step losses.
-
Direct nitration of fluorinated precursors : Yields up to 85% but requires high-purity starting materials.
-
Catalytic dechlorination : Yields exceed 90% but demands specialized equipment for H₂ handling.
Purity and Byproduct Formation
Byproducts like 2,3,4,5-tetrachlorofluorobenzene (up to 10% in some cases ) necessitate rigorous purification. Gas chromatography (GC) and nuclear magnetic resonance (NMR) are standard for quality control.
Industrial-Scale Production Considerations
Scalability Challenges
Large-scale reactions face heat dissipation issues during exothermic nitration. Continuous-flow reactors mitigate this by enabling precise temperature control . Additionally, catalyst recovery and reuse are critical for cost-effectiveness.
Chemical Reactions Analysis
Types of Reactions
1,3-Difluoro-5-nitrobenzene undergoes various chemical reactions, including:
Nucleophilic Substitution: The presence of electron-withdrawing fluorine and nitro groups makes the compound susceptible to nucleophilic aromatic substitution reactions. Common nucleophiles include amines and alkoxides.
Reduction: The nitro group can be reduced to an amino group using reducing agents such as hydrogen gas in the presence of a catalyst or metal hydrides.
Oxidation: Although less common, the compound can undergo oxidation reactions under specific conditions.
Common Reagents and Conditions
Nucleophilic Substitution: Reagents like sodium methoxide or potassium tert-butoxide in polar aprotic solvents (e.g., dimethyl sulfoxide) are commonly used.
Reduction: Catalysts such as palladium on carbon (Pd/C) or platinum oxide (PtO₂) are employed in hydrogenation reactions.
Oxidation: Strong oxidizing agents like potassium permanganate (KMnO₄) or chromium trioxide (CrO₃) can be used under controlled conditions.
Major Products Formed
Nucleophilic Substitution: Products include substituted benzene derivatives where the fluorine atoms are replaced by nucleophiles.
Reduction: The primary product is 1,3-difluoro-5-aminobenzene.
Oxidation: Depending on the conditions, various oxidized products can be formed, though these reactions are less common.
Scientific Research Applications
Synthesis Pathways
3,5-Difluoronitrobenzene can be synthesized through various methods, including halogenation and nitration processes. A notable synthesis involves the reaction of 2,4,5-trichloronitrobenzene with alkali metal fluorides under specific conditions to yield 3,5-difluoroaniline, which is crucial for further applications in pharmaceuticals and agrochemicals .
Pharmaceutical Applications
This compound serves as an important intermediate in the synthesis of various pharmaceutical compounds. Its derivatives are utilized in the development of drugs due to their enhanced biological activity and specificity. For instance:
- Anticancer Agents : Research indicates that derivatives of 3,5-DFNB exhibit cytotoxic properties against certain cancer cell lines .
- Antimicrobial Agents : Compounds synthesized from 3,5-DFNB have demonstrated significant antimicrobial activity, making them potential candidates for new antibiotics .
Agrochemical Applications
In agrochemistry, this compound is used as an intermediate in the synthesis of pesticides. For example:
- Teflubenzuron : This compound is synthesized using this compound as a precursor. Teflubenzuron is an effective insect growth regulator that targets pests in agricultural settings .
Material Science Applications
Recent studies have explored the use of this compound in enhancing the thermal stability of enzymes. For instance:
- Immobilized Porcine Pancreas Lipase : The incorporation of 3,5-DFNB has been shown to improve the thermal stability and activity of this enzyme, which is crucial for various biotechnological applications .
Case Study 1: Synthesis of Anticancer Agents
A study published in the Journal of Organic Chemistry detailed the synthesis of novel anticancer agents derived from this compound. The researchers reported improved efficacy compared to existing treatments through structure-activity relationship (SAR) studies .
Case Study 2: Development of Teflubenzuron
Research highlighted a synthetic pathway for teflubenzuron involving this compound as a key intermediate. The study emphasized the compound's role in increasing the yield and purity of the final product .
Data Table: Applications Summary
Mechanism of Action
The mechanism of action of 1,3-difluoro-5-nitrobenzene involves its interaction with molecular targets through its functional groups. The nitro group can participate in redox reactions, while the fluorine atoms influence the compound’s electronic properties. These interactions can affect various biochemical pathways, making the compound useful in studying enzyme inhibition and receptor binding.
Comparison with Similar Compounds
Comparison with Similar Difluoronitrobenzene Isomers
Structural and Electronic Properties
The reactivity and applications of difluoronitrobenzenes are highly dependent on the positions of fluorine and nitro groups. Key isomers include:
Electronic Effects :
- The 3,5-isomer exhibits symmetric electron withdrawal, leading to predictable reactivity in cross-coupling reactions (e.g., 82% yield with 1,3-dimethoxybenzene in 5 days) .
- The 2,4-isomer shows enhanced activation for nucleophilic aromatic substitution (SNAr) due to fluorine's ortho/para-directing effects, enabling efficient synthesis of ortho-substituted morpholine derivatives .
Reactivity in Cross-Coupling Reactions
This compound participates in copper-catalyzed cross-couplings but requires longer reaction times compared to other isomers. For example:
- Coupling with 1,3-dimethoxybenzene takes 5 days for 82% yield .
- In contrast, 2,4-dimethoxyiodobenzene reacts completely in 12 hours under similar conditions, highlighting the role of iodination efficiency and electronic effects .
Inhibition Mechanisms : Residual iodine in reactions involving this compound can oxidize Cu(I) to inactive Cu(II), slowing catalysis. This is mitigated by optimizing iodination steps .
Biological Activity
3,5-Difluoronitrobenzene (DFNB) is a fluorinated aromatic compound that has garnered attention in various fields of research, particularly in medicinal chemistry and biochemistry. This article explores its biological activity, mechanisms of action, and relevant case studies, while providing a comprehensive overview of its applications and implications.
The biological activity of DFNB primarily arises from its ability to undergo various chemical reactions, including:
- Nucleophilic Aromatic Substitution : DFNB can participate in nucleophilic aromatic substitution reactions, where nucleophiles replace the nitro or fluorine groups on the benzene ring.
- Reduction : The nitro group can be reduced to an amine, potentially leading to reactive intermediates that interact with biological macromolecules.
- Oxidation : Under specific conditions, DFNB may be oxidized to form different products that could exhibit varying biological activities.
Biological Applications
DFNB serves as an important intermediate in the synthesis of pharmaceuticals and agrochemicals. Its derivatives have been studied for their potential antibacterial and anticancer activities.
Antibacterial Activity
A study on oxazolidinone antibiotics demonstrated that analogs derived from DFNB exhibited significant antibacterial properties. These compounds inhibit bacterial protein synthesis by binding to the 50S ribosomal subunit, which is crucial for translation . The following table summarizes the antibacterial activity of selected DFNB derivatives:
Compound | Activity (MIC µg/mL) | Target Bacteria |
---|---|---|
Oxazolidinone derivative 1 | 8 | Staphylococcus aureus |
Oxazolidinone derivative 2 | 16 | Escherichia coli |
Oxazolidinone derivative 3 | 32 | Pseudomonas aeruginosa |
Case Studies
-
Synthesis of 3,5-Difluoroaniline :
A notable process involves converting DFNB to 3,5-difluoroaniline through reduction reactions. This compound is essential in producing various industrial chemicals and pharmaceuticals . -
Metabolism Studies :
Research into the metabolic pathways of DFNB revealed significant differences in biotransformation patterns when compared to its analogs. Studies using F NMR analysis demonstrated distinct metabolite profiles that could influence its biological effects . -
Electron Attachment Studies :
Investigations into the electron attachment properties of DFNB indicated both non-dissociative and dissociative pathways. These findings provide insights into its reactivity under physiological conditions, potentially affecting its biological interactions .
Q & A
Basic Questions
Q. What are the common synthetic routes for 3,5-Difluoronitrobenzene, and what analytical methods validate its purity?
- Answer : this compound is typically synthesized via nitration or fluorination of substituted benzene derivatives. For example, fluorination of nitrobenzene precursors using hydrogen fluoride under controlled conditions can yield the product . Validation of purity involves chromatographic techniques (e.g., HPLC for quantifying reaction intermediates and byproducts) and spectroscopic methods (e.g., NMR for structural confirmation). Gas chromatography-mass spectrometry (GC-MS) and elemental analysis are also employed to ensure >99% purity .
Q. How do substituent positions influence the molecular conformation of difluoronitrobenzenes?
- Answer : Substituent positions significantly affect molecular geometry. For this compound (C₂v symmetry), the nitro group remains planar with the aromatic ring, while steric hindrance in 2,6-difluoronitrobenzene forces the nitro group to rotate out of the plane (torsional angle ~54°) . Gas-phase electron diffraction (GED) and quantum chemical calculations (MP2, B3LYP) reveal bond length variations: C-F bonds are shorter in meta positions (1.347 Å) compared to ortho isomers (1.344 Å) .
Q. What safety precautions are essential when handling this compound in laboratory settings?
- Answer : Due to its nitroaromatic nature, this compound requires handling in a fume hood with PPE (gloves, goggles, lab coat). It should be stored away from reducing agents to prevent explosive reactions. Waste disposal must follow hazardous chemical protocols. Institutional guidelines for handling "research-use-only" compounds, as outlined in safety data sheets, should be strictly followed .
Advanced Research Questions
Q. How can copper-catalyzed cross-coupling reactions involving 3,5-DFNB be optimized to minimize reaction times?
- Answer : Reaction efficiency depends on iodination kinetics and catalyst stability. For example, coupling 3,5-DFNB with electron-rich arenes (e.g., 1,3-dimethoxybenzene) requires 5 days for 82% yield, but pre-iodination of one substrate reduces time to 12 hours . Inhibitors like residual iodine (I₂) oxidize Cu(I) to inactive Cu(II), slowing reactions. Adding LiI (0.5 equiv) mitigates this by scavenging free iodine, restoring catalytic activity .
Q. What role does Design of Experiments (DoE) play in optimizing continuous flow synthesis parameters for reactions involving 3,5-DFNB?
- Answer : DoE identifies critical factors (residence time, temperature, reagent equivalents) and their interactions. For instance, a three-factor DoE (residence time: 0.5–3.5 min, temperature: 30–70°C, pyrrolidine equivalents: 2–10) optimizes nucleophilic aromatic substitution in flow reactors. HPLC quantifies product distribution, while MODDE Pro software models non-linear relationships between variables .
Q. How do computational methods like MP2 and B3LYP contribute to understanding the rotational barriers of the nitro group in 3,5-DFNB?
- Answer : B3LYP/cc-pVTZ calculations predict a nitro group rotation barrier (V₉₀) of ~10 kJ/mol, but GED experiments show a lower experimental value (4 kJ/mol). This discrepancy arises from anharmonic vibrational corrections not fully accounted for in static computational models . Dynamic GED refinements incorporating torsional motion align better with observed barriers .
Q. What are the implications of conflicting experimental and computational data on the internal rotation barriers of the nitro group in 3,5-DFNB?
- Answer : The lower experimental barrier suggests computational models may overestimate steric or electronic effects. Hybrid methods combining GED with molecular dynamics (MD) simulations could resolve this by modeling temperature-dependent conformational flexibility. Such contradictions highlight the need for multi-method validation in structural studies .
Q. How can QSAR models predict the toxicity profile of this compound based on its electronic and structural properties?
- Answer : Quantitative Structure-Activity Relationship (QSAR) models use descriptors like Hammett constants (σ), dipole moments, and frontier orbital energies to predict toxicity. For nitroaromatics, electron-withdrawing groups (e.g., -NO₂, -F) increase electrophilicity, correlating with higher cytotoxicity. Genetic algorithm-based QSAR analyses on analogues (e.g., 2,5-difluoronitrobenzene) identify metabolic pathways involving nitro-reduction as key toxicity drivers .
Properties
IUPAC Name |
1,3-difluoro-5-nitrobenzene | |
---|---|---|
Source | PubChem | |
URL | https://pubchem.ncbi.nlm.nih.gov | |
Description | Data deposited in or computed by PubChem | |
InChI |
InChI=1S/C6H3F2NO2/c7-4-1-5(8)3-6(2-4)9(10)11/h1-3H | |
Source | PubChem | |
URL | https://pubchem.ncbi.nlm.nih.gov | |
Description | Data deposited in or computed by PubChem | |
InChI Key |
AUQBBDWDLJSKMI-UHFFFAOYSA-N | |
Source | PubChem | |
URL | https://pubchem.ncbi.nlm.nih.gov | |
Description | Data deposited in or computed by PubChem | |
Canonical SMILES |
C1=C(C=C(C=C1F)F)[N+](=O)[O-] | |
Source | PubChem | |
URL | https://pubchem.ncbi.nlm.nih.gov | |
Description | Data deposited in or computed by PubChem | |
Molecular Formula |
C6H3F2NO2 | |
Source | PubChem | |
URL | https://pubchem.ncbi.nlm.nih.gov | |
Description | Data deposited in or computed by PubChem | |
DSSTOX Substance ID |
DTXSID30177193 | |
Record name | 1,3-Difluoro-5-nitrobenzene | |
Source | EPA DSSTox | |
URL | https://comptox.epa.gov/dashboard/DTXSID30177193 | |
Description | DSSTox provides a high quality public chemistry resource for supporting improved predictive toxicology. | |
Molecular Weight |
159.09 g/mol | |
Source | PubChem | |
URL | https://pubchem.ncbi.nlm.nih.gov | |
Description | Data deposited in or computed by PubChem | |
CAS No. |
2265-94-3 | |
Record name | 1,3-Difluoro-5-nitrobenzene | |
Source | CAS Common Chemistry | |
URL | https://commonchemistry.cas.org/detail?cas_rn=2265-94-3 | |
Description | CAS Common Chemistry is an open community resource for accessing chemical information. Nearly 500,000 chemical substances from CAS REGISTRY cover areas of community interest, including common and frequently regulated chemicals, and those relevant to high school and undergraduate chemistry classes. This chemical information, curated by our expert scientists, is provided in alignment with our mission as a division of the American Chemical Society. | |
Explanation | The data from CAS Common Chemistry is provided under a CC-BY-NC 4.0 license, unless otherwise stated. | |
Record name | 1,3-Difluoro-5-nitrobenzene | |
Source | ChemIDplus | |
URL | https://pubchem.ncbi.nlm.nih.gov/substance/?source=chemidplus&sourceid=0002265943 | |
Description | ChemIDplus is a free, web search system that provides access to the structure and nomenclature authority files used for the identification of chemical substances cited in National Library of Medicine (NLM) databases, including the TOXNET system. | |
Record name | 2265-94-3 | |
Source | DTP/NCI | |
URL | https://dtp.cancer.gov/dtpstandard/servlet/dwindex?searchtype=NSC&outputformat=html&searchlist=10260 | |
Description | The NCI Development Therapeutics Program (DTP) provides services and resources to the academic and private-sector research communities worldwide to facilitate the discovery and development of new cancer therapeutic agents. | |
Explanation | Unless otherwise indicated, all text within NCI products is free of copyright and may be reused without our permission. Credit the National Cancer Institute as the source. | |
Record name | 1,3-Difluoro-5-nitrobenzene | |
Source | EPA DSSTox | |
URL | https://comptox.epa.gov/dashboard/DTXSID30177193 | |
Description | DSSTox provides a high quality public chemistry resource for supporting improved predictive toxicology. | |
Record name | 1,3-difluoro-5-nitrobenzene | |
Source | European Chemicals Agency (ECHA) | |
URL | https://echa.europa.eu/substance-information/-/substanceinfo/100.017.153 | |
Description | The European Chemicals Agency (ECHA) is an agency of the European Union which is the driving force among regulatory authorities in implementing the EU's groundbreaking chemicals legislation for the benefit of human health and the environment as well as for innovation and competitiveness. | |
Explanation | Use of the information, documents and data from the ECHA website is subject to the terms and conditions of this Legal Notice, and subject to other binding limitations provided for under applicable law, the information, documents and data made available on the ECHA website may be reproduced, distributed and/or used, totally or in part, for non-commercial purposes provided that ECHA is acknowledged as the source: "Source: European Chemicals Agency, http://echa.europa.eu/". Such acknowledgement must be included in each copy of the material. ECHA permits and encourages organisations and individuals to create links to the ECHA website under the following cumulative conditions: Links can only be made to webpages that provide a link to the Legal Notice page. | |
Record name | 1,3-DIFLUORO-5-NITROBENZENE | |
Source | FDA Global Substance Registration System (GSRS) | |
URL | https://gsrs.ncats.nih.gov/ginas/app/beta/substances/27ZN24954J | |
Description | The FDA Global Substance Registration System (GSRS) enables the efficient and accurate exchange of information on what substances are in regulated products. Instead of relying on names, which vary across regulatory domains, countries, and regions, the GSRS knowledge base makes it possible for substances to be defined by standardized, scientific descriptions. | |
Explanation | Unless otherwise noted, the contents of the FDA website (www.fda.gov), both text and graphics, are not copyrighted. They are in the public domain and may be republished, reprinted and otherwise used freely by anyone without the need to obtain permission from FDA. Credit to the U.S. Food and Drug Administration as the source is appreciated but not required. | |
Synthesis routes and methods
Procedure details
Retrosynthesis Analysis
AI-Powered Synthesis Planning: Our tool employs the Template_relevance Pistachio, Template_relevance Bkms_metabolic, Template_relevance Pistachio_ringbreaker, Template_relevance Reaxys, Template_relevance Reaxys_biocatalysis model, leveraging a vast database of chemical reactions to predict feasible synthetic routes.
One-Step Synthesis Focus: Specifically designed for one-step synthesis, it provides concise and direct routes for your target compounds, streamlining the synthesis process.
Accurate Predictions: Utilizing the extensive PISTACHIO, BKMS_METABOLIC, PISTACHIO_RINGBREAKER, REAXYS, REAXYS_BIOCATALYSIS database, our tool offers high-accuracy predictions, reflecting the latest in chemical research and data.
Strategy Settings
Precursor scoring | Relevance Heuristic |
---|---|
Min. plausibility | 0.01 |
Model | Template_relevance |
Template Set | Pistachio/Bkms_metabolic/Pistachio_ringbreaker/Reaxys/Reaxys_biocatalysis |
Top-N result to add to graph | 6 |
Feasible Synthetic Routes
Disclaimer and Information on In-Vitro Research Products
Please be aware that all articles and product information presented on BenchChem are intended solely for informational purposes. The products available for purchase on BenchChem are specifically designed for in-vitro studies, which are conducted outside of living organisms. In-vitro studies, derived from the Latin term "in glass," involve experiments performed in controlled laboratory settings using cells or tissues. It is important to note that these products are not categorized as medicines or drugs, and they have not received approval from the FDA for the prevention, treatment, or cure of any medical condition, ailment, or disease. We must emphasize that any form of bodily introduction of these products into humans or animals is strictly prohibited by law. It is essential to adhere to these guidelines to ensure compliance with legal and ethical standards in research and experimentation.