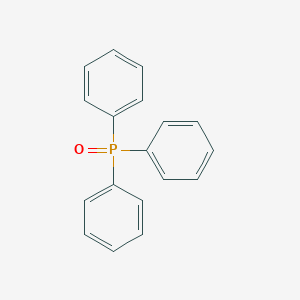
Triphenylphosphine oxide
Overview
Description
Triphenylphosphine oxide is an organophosphorus compound with the chemical formula OP(C₆H₅)₃. It is a colorless crystalline solid that is commonly produced as a byproduct in reactions involving triphenylphosphine. This compound is known for its stability and is widely used in various chemical processes and research applications .
Mechanism of Action
Target of Action
Triphenylphosphine oxide (TPPO) is an organophosphorus compound . It doesn’t have a specific biological target because it’s primarily used as a reagent in organic synthesis . It’s known to interact with various organic compounds, particularly those that have acidic hydrogen atoms, such as phenols .
Mode of Action
TPPO is a byproduct of many useful reactions in organic synthesis, including the Wittig, Staudinger, and Mitsunobu reactions . It’s also formed when PPh3Cl2 is employed to convert alcohols into alkyl chlorides . The oxygen center in TPPO is relatively basic, which makes it a popular agent to crystallize otherwise difficult-to-crystallize molecules .
Biochemical Pathways
As a reagent and byproduct in organic synthesis, TPPO is involved in various biochemical pathways. For instance, in the Wittig reaction, it’s produced as a stable and stoichiometric by-product . The reaction is crucial in organic synthesis for the preparation of alkenes .
Pharmacokinetics
TPPO is slightly soluble in water but more soluble in polar organic solvents . These properties can affect its distribution and elimination if it were to be used in a biological system.
Result of Action
The primary result of TPPO’s action in organic synthesis reactions is the formation of new compounds. For example, in the Wittig reaction, it helps in the formation of alkenes . Additionally, due to its basic oxygen center and the rigidity of its backbone, TPPO can induce the crystallization of chemical compounds .
Action Environment
The action of TPPO can be influenced by various environmental factors. For instance, its solubility can be affected by the polarity of the solvent, which can impact its efficacy in reactions . Furthermore, TPPO’s stability and reactivity can be influenced by temperature and the presence of other reactive species .
Biochemical Analysis
Biochemical Properties
Triphenylphosphine oxide plays a significant role in biochemical reactions, particularly in the context of organic synthesis. It is known to interact with various enzymes, proteins, and other biomolecules. One of the key interactions of this compound is with acidic hydrogen atoms, such as those found in phenols. The basicity of the oxygen center in this compound allows it to form stable complexes with these molecules, facilitating crystallization and purification processes .
In addition, this compound is involved in the Wittig, Staudinger, and Mitsunobu reactions, where it acts as a byproduct. These reactions are crucial in the synthesis of various organic compounds, and the presence of this compound can influence the overall yield and purity of the final products .
Cellular Effects
This compound has been shown to affect various types of cells and cellular processes. It influences cell function by interacting with cell signaling pathways, gene expression, and cellular metabolism. For instance, this compound has been identified as a potent and selective inhibitor of the transient receptor potential melastatin-5 ion channel, which plays a critical role in taste pathways and insulin secretion . This inhibition can lead to changes in cellular signaling and metabolic processes, affecting overall cell function.
Molecular Mechanism
The molecular mechanism of this compound involves its ability to form stable complexes with various biomolecules. The oxygen center in this compound is relatively basic, allowing it to interact with acidic hydrogen atoms and form hydrogen bonds. This interaction is crucial in the crystallization of difficult-to-crystallize molecules .
Furthermore, this compound acts as an inhibitor of the transient receptor potential melastatin-5 ion channel by binding to the channel and preventing its activation. This inhibition affects the downstream signaling pathways and cellular responses associated with the ion channel .
Temporal Effects in Laboratory Settings
In laboratory settings, the effects of this compound can change over time. The stability and degradation of this compound are important factors to consider in experimental setups. This compound is relatively stable under standard laboratory conditions, but its solubility in various solvents can affect its long-term behavior. For example, this compound is poorly soluble in hexane and cold diethyl ether, which can influence its separation and purification in chemical reactions .
Dosage Effects in Animal Models
The effects of this compound vary with different dosages in animal models. Studies have shown that this compound can have toxic effects at high doses. For instance, exposure to high concentrations of this compound has been associated with changes in cholinesterase activity levels in the brain and plasma of animal models . These findings highlight the importance of careful dosage control in experimental and therapeutic applications.
Metabolic Pathways
This compound is involved in various metabolic pathways, particularly those related to its role as an inhibitor of the transient receptor potential melastatin-5 ion channel. This inhibition affects the signaling pathways and metabolic processes regulated by the ion channel, leading to changes in metabolic flux and metabolite levels . Additionally, this compound can be regenerated from its oxidized form through reactions with reducing agents such as trichlorosilane .
Transport and Distribution
The transport and distribution of this compound within cells and tissues are influenced by its interactions with transporters and binding proteins. This compound can form complexes with various biomolecules, affecting its localization and accumulation within cells. Its poor solubility in certain solvents can also impact its distribution in biological systems .
Subcellular Localization
This compound is localized in specific subcellular compartments based on its interactions with biomolecules and its chemical properties. The basicity of the oxygen center in this compound allows it to interact with acidic hydrogen atoms, directing it to specific cellular compartments where these interactions are favorable . This localization can influence the activity and function of this compound within cells.
Preparation Methods
Synthetic Routes and Reaction Conditions: Triphenylphosphine oxide can be synthesized through several methods. One common method involves the oxidation of triphenylphosphine using hydrogen peroxide or other oxidizing agents. The reaction is typically carried out in an organic solvent such as dichloromethane at room temperature .
Industrial Production Methods: In industrial settings, this compound is often produced as a byproduct in large-scale reactions such as the Wittig, Staudinger, and Mitsunobu reactions. These reactions involve the use of triphenylphosphine as a reagent, and the oxide is formed as a result of the oxidation of triphenylphosphine during the reaction process .
Chemical Reactions Analysis
Types of Reactions: Triphenylphosphine oxide undergoes various chemical reactions, including:
Oxidation: It can be further oxidized to form other phosphorus-containing compounds.
Reduction: It can be reduced back to triphenylphosphine using reducing agents such as trichlorosilane or phosgene.
Substitution: It can participate in substitution reactions where the oxygen atom is replaced by other functional groups.
Common Reagents and Conditions:
Oxidation: Hydrogen peroxide, dichloromethane, room temperature.
Reduction: Trichlorosilane, phosgene, triethylamine.
Major Products:
Oxidation: Formation of higher oxidation state phosphorus compounds.
Reduction: Regeneration of triphenylphosphine.
Scientific Research Applications
Triphenylphosphine oxide has a wide range of applications in scientific research:
Chemistry: It is used as a ligand in coordination chemistry and as a reagent in various organic synthesis reactions.
Biology: It is used in the study of enzyme mechanisms and as a stabilizing agent for proteins.
Medicine: It is used in the development of pharmaceuticals and as a catalyst in drug synthesis.
Industry: It is used in the production of polymers, resins, and other industrial chemicals.
Comparison with Similar Compounds
Triphenylphosphine: Similar in structure but lacks the oxygen atom.
Phosphoryl chloride: Contains a phosphorus-oxygen bond but has different reactivity and applications.
Phosphorus pentachloride: Another phosphorus-containing compound with different chemical properties.
Uniqueness: Triphenylphosphine oxide is unique due to its stability and versatility in various chemical reactions. Its ability to act as a ligand and form coordination complexes makes it valuable in both research and industrial applications .
Biological Activity
Triphenylphosphine oxide (TPPO), a prominent organophosphorus compound, has garnered attention due to its diverse biological activities and applications in various fields, including organic synthesis, catalysis, and materials science. This article provides a comprehensive overview of the biological activity of TPPO, supported by data tables, case studies, and detailed research findings.
This compound is represented by the formula OP(C₆H₅)₃ and is characterized by a tetrahedral geometry around the phosphorus atom. The P-O bond length is approximately 1.48 Å, indicating a strong covalent bond that contributes to its stability and reactivity in biological systems . The compound is often used as a reagent in organic synthesis due to its ability to facilitate crystallization processes and its role as a byproduct in several reactions, such as the Wittig and Staudinger reactions .
Antimicrobial Properties
TPPO has demonstrated notable antimicrobial activity against various pathogens. In a study evaluating its efficacy against bacterial strains, TPPO exhibited significant inhibition of growth for both Gram-positive and Gram-negative bacteria. The minimum inhibitory concentration (MIC) values ranged from 50 to 200 µg/mL, depending on the bacterial strain tested .
Table 1: Antimicrobial Activity of TPPO
Bacterial Strain | MIC (µg/mL) |
---|---|
Staphylococcus aureus | 50 |
Escherichia coli | 100 |
Pseudomonas aeruginosa | 200 |
Cytotoxic Effects
Research has indicated that TPPO exhibits cytotoxic effects on certain cancer cell lines. In vitro studies have shown that TPPO can induce apoptosis in human breast cancer cells (MCF-7) at concentrations above 100 µM. The mechanism appears to involve the generation of reactive oxygen species (ROS) leading to oxidative stress and subsequent cell death .
Case Study: Cytotoxicity in MCF-7 Cells
A study conducted by Zhang et al. (2020) explored the effects of TPPO on MCF-7 cells. The results indicated:
- Concentration : 100 µM TPPO
- Duration : 24 hours
- Outcome : Induction of apoptosis confirmed by flow cytometry analysis.
Role in Enzyme Inhibition
TPPO has also been investigated for its potential as an enzyme inhibitor. It has been shown to inhibit acetylcholinesterase (AChE), an enzyme critical for neurotransmission. This inhibition could have implications for neurodegenerative diseases such as Alzheimer's disease. The IC₅₀ value for AChE inhibition was reported to be approximately 75 µM .
Applications in Synthesis and Catalysis
In addition to its biological activities, TPPO serves as a versatile catalyst in organic synthesis. It facilitates various reactions, including:
Properties
IUPAC Name |
diphenylphosphorylbenzene | |
---|---|---|
Source | PubChem | |
URL | https://pubchem.ncbi.nlm.nih.gov | |
Description | Data deposited in or computed by PubChem | |
InChI |
InChI=1S/C18H15OP/c19-20(16-10-4-1-5-11-16,17-12-6-2-7-13-17)18-14-8-3-9-15-18/h1-15H | |
Source | PubChem | |
URL | https://pubchem.ncbi.nlm.nih.gov | |
Description | Data deposited in or computed by PubChem | |
InChI Key |
FIQMHBFVRAXMOP-UHFFFAOYSA-N | |
Source | PubChem | |
URL | https://pubchem.ncbi.nlm.nih.gov | |
Description | Data deposited in or computed by PubChem | |
Canonical SMILES |
C1=CC=C(C=C1)P(=O)(C2=CC=CC=C2)C3=CC=CC=C3 | |
Source | PubChem | |
URL | https://pubchem.ncbi.nlm.nih.gov | |
Description | Data deposited in or computed by PubChem | |
Molecular Formula |
C18H15OP | |
Source | PubChem | |
URL | https://pubchem.ncbi.nlm.nih.gov | |
Description | Data deposited in or computed by PubChem | |
DSSTOX Substance ID |
DTXSID2022121 | |
Record name | Triphenylphosphine oxide | |
Source | EPA DSSTox | |
URL | https://comptox.epa.gov/dashboard/DTXSID2022121 | |
Description | DSSTox provides a high quality public chemistry resource for supporting improved predictive toxicology. | |
Molecular Weight |
278.3 g/mol | |
Source | PubChem | |
URL | https://pubchem.ncbi.nlm.nih.gov | |
Description | Data deposited in or computed by PubChem | |
Physical Description |
Solid; [IUCLID] Beige crystalline solid; [Aldrich MSDS] | |
Record name | Triphenylphosphine oxide | |
Source | Haz-Map, Information on Hazardous Chemicals and Occupational Diseases | |
URL | https://haz-map.com/Agents/9546 | |
Description | Haz-Map® is an occupational health database designed for health and safety professionals and for consumers seeking information about the adverse effects of workplace exposures to chemical and biological agents. | |
Explanation | Copyright (c) 2022 Haz-Map(R). All rights reserved. Unless otherwise indicated, all materials from Haz-Map are copyrighted by Haz-Map(R). No part of these materials, either text or image may be used for any purpose other than for personal use. Therefore, reproduction, modification, storage in a retrieval system or retransmission, in any form or by any means, electronic, mechanical or otherwise, for reasons other than personal use, is strictly prohibited without prior written permission. | |
CAS No. |
791-28-6 | |
Record name | Triphenylphosphine oxide | |
Source | CAS Common Chemistry | |
URL | https://commonchemistry.cas.org/detail?cas_rn=791-28-6 | |
Description | CAS Common Chemistry is an open community resource for accessing chemical information. Nearly 500,000 chemical substances from CAS REGISTRY cover areas of community interest, including common and frequently regulated chemicals, and those relevant to high school and undergraduate chemistry classes. This chemical information, curated by our expert scientists, is provided in alignment with our mission as a division of the American Chemical Society. | |
Explanation | The data from CAS Common Chemistry is provided under a CC-BY-NC 4.0 license, unless otherwise stated. | |
Record name | Triphenylphosphine oxide | |
Source | ChemIDplus | |
URL | https://pubchem.ncbi.nlm.nih.gov/substance/?source=chemidplus&sourceid=0000791286 | |
Description | ChemIDplus is a free, web search system that provides access to the structure and nomenclature authority files used for the identification of chemical substances cited in National Library of Medicine (NLM) databases, including the TOXNET system. | |
Record name | TRIPHENYLPHOSPHINE OXIDE | |
Source | DTP/NCI | |
URL | https://dtp.cancer.gov/dtpstandard/servlet/dwindex?searchtype=NSC&outputformat=html&searchlist=398 | |
Description | The NCI Development Therapeutics Program (DTP) provides services and resources to the academic and private-sector research communities worldwide to facilitate the discovery and development of new cancer therapeutic agents. | |
Explanation | Unless otherwise indicated, all text within NCI products is free of copyright and may be reused without our permission. Credit the National Cancer Institute as the source. | |
Record name | Phosphine oxide, triphenyl- | |
Source | EPA Chemicals under the TSCA | |
URL | https://www.epa.gov/chemicals-under-tsca | |
Description | EPA Chemicals under the Toxic Substances Control Act (TSCA) collection contains information on chemicals and their regulations under TSCA, including non-confidential content from the TSCA Chemical Substance Inventory and Chemical Data Reporting. | |
Record name | Triphenylphosphine oxide | |
Source | EPA DSSTox | |
URL | https://comptox.epa.gov/dashboard/DTXSID2022121 | |
Description | DSSTox provides a high quality public chemistry resource for supporting improved predictive toxicology. | |
Record name | Triphenylphosphine oxide | |
Source | European Chemicals Agency (ECHA) | |
URL | https://echa.europa.eu/substance-information/-/substanceinfo/100.011.217 | |
Description | The European Chemicals Agency (ECHA) is an agency of the European Union which is the driving force among regulatory authorities in implementing the EU's groundbreaking chemicals legislation for the benefit of human health and the environment as well as for innovation and competitiveness. | |
Explanation | Use of the information, documents and data from the ECHA website is subject to the terms and conditions of this Legal Notice, and subject to other binding limitations provided for under applicable law, the information, documents and data made available on the ECHA website may be reproduced, distributed and/or used, totally or in part, for non-commercial purposes provided that ECHA is acknowledged as the source: "Source: European Chemicals Agency, http://echa.europa.eu/". Such acknowledgement must be included in each copy of the material. ECHA permits and encourages organisations and individuals to create links to the ECHA website under the following cumulative conditions: Links can only be made to webpages that provide a link to the Legal Notice page. | |
Record name | TRIPHENYLPHOSPHINE OXIDE | |
Source | FDA Global Substance Registration System (GSRS) | |
URL | https://gsrs.ncats.nih.gov/ginas/app/beta/substances/Z69161FKI3 | |
Description | The FDA Global Substance Registration System (GSRS) enables the efficient and accurate exchange of information on what substances are in regulated products. Instead of relying on names, which vary across regulatory domains, countries, and regions, the GSRS knowledge base makes it possible for substances to be defined by standardized, scientific descriptions. | |
Explanation | Unless otherwise noted, the contents of the FDA website (www.fda.gov), both text and graphics, are not copyrighted. They are in the public domain and may be republished, reprinted and otherwise used freely by anyone without the need to obtain permission from FDA. Credit to the U.S. Food and Drug Administration as the source is appreciated but not required. | |
Synthesis routes and methods I
Procedure details
Synthesis routes and methods II
Procedure details
Synthesis routes and methods III
Procedure details
Synthesis routes and methods IV
Procedure details
Synthesis routes and methods V
Procedure details
Retrosynthesis Analysis
AI-Powered Synthesis Planning: Our tool employs the Template_relevance Pistachio, Template_relevance Bkms_metabolic, Template_relevance Pistachio_ringbreaker, Template_relevance Reaxys, Template_relevance Reaxys_biocatalysis model, leveraging a vast database of chemical reactions to predict feasible synthetic routes.
One-Step Synthesis Focus: Specifically designed for one-step synthesis, it provides concise and direct routes for your target compounds, streamlining the synthesis process.
Accurate Predictions: Utilizing the extensive PISTACHIO, BKMS_METABOLIC, PISTACHIO_RINGBREAKER, REAXYS, REAXYS_BIOCATALYSIS database, our tool offers high-accuracy predictions, reflecting the latest in chemical research and data.
Strategy Settings
Precursor scoring | Relevance Heuristic |
---|---|
Min. plausibility | 0.01 |
Model | Template_relevance |
Template Set | Pistachio/Bkms_metabolic/Pistachio_ringbreaker/Reaxys/Reaxys_biocatalysis |
Top-N result to add to graph | 6 |
Feasible Synthetic Routes
Disclaimer and Information on In-Vitro Research Products
Please be aware that all articles and product information presented on BenchChem are intended solely for informational purposes. The products available for purchase on BenchChem are specifically designed for in-vitro studies, which are conducted outside of living organisms. In-vitro studies, derived from the Latin term "in glass," involve experiments performed in controlled laboratory settings using cells or tissues. It is important to note that these products are not categorized as medicines or drugs, and they have not received approval from the FDA for the prevention, treatment, or cure of any medical condition, ailment, or disease. We must emphasize that any form of bodily introduction of these products into humans or animals is strictly prohibited by law. It is essential to adhere to these guidelines to ensure compliance with legal and ethical standards in research and experimentation.