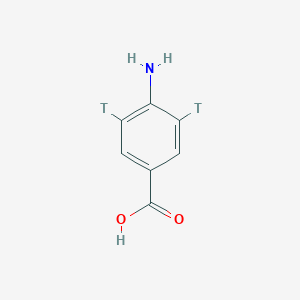
4-Amino-3,5-ditritiobenzoic acid
Overview
Description
4-Amino-3,5-dichlorobenzoic acid (CAS 56961-25-2) is an aromatic compound with the molecular formula C₇H₅Cl₂NO₂. It features a benzoic acid backbone substituted with an amino group at the para position and chlorine atoms at the meta positions (3 and 5). This structural arrangement confers unique electronic and steric properties, making it valuable in organic synthesis, pharmaceutical research, and materials science. The amino group facilitates nucleophilic reactions (e.g., amide bond formation), while the chlorine substituents enhance the compound’s acidity and influence its reactivity in electrophilic aromatic substitutions .
Key applications include:
- Pharmaceutical intermediates: Used in synthesizing active pharmaceutical ingredients (APIs) due to its reactive amino and carboxylic acid groups .
- Dye and polymer synthesis: Serves as a precursor for halogenated aromatic dyes and specialty polymers .
- Mechanistic studies: Employed to investigate halogenation and substitution reactions in aromatic systems .
Preparation Methods
Synthetic Route Design and Mechanistic Considerations
Nitration of 4-Chlorobenzoic Acid
The initial step involves the nitration of 4-chlorobenzoic acid to form 4-chloro-3,5-dinitrobenzoic acid. This reaction proceeds via electrophilic aromatic substitution, where the electron-withdrawing chloro and carboxylic acid groups direct nitro groups to the meta positions. Concentrated sulfuric acid acts as both a catalyst and dehydrating agent, facilitating the generation of nitronium ions (NO₂⁺) from nitric acid .
Key Reaction Parameters
Parameter | Value |
---|---|
Nitrating agent | Fuming HNO₃ (5 eq) |
Catalyst | H₂SO₄ (conc.) |
Temperature | 125°C |
Duration | 2 hours |
Yield | 89% |
The stoichiometric excess of nitric acid ensures complete di-nitration, while elevated temperatures (125°C) accelerate the reaction kinetics. Post-reaction, the product precipitates upon ice quenching, enabling straightforward isolation .
Amination of 4-Chloro-3,5-Dinitrobenzoic Acid
The chloro substituent is replaced by an amino group via nucleophilic aromatic substitution (NAS) using aqueous ammonia in methanol. The electron-deficient aromatic ring facilitates attack by ammonia, with the carboxylic acid group enhancing ring activation through resonance withdrawal .
Optimized Amination Conditions
Parameter | Value |
---|---|
Solvent | Methanol |
Ammonia concentration | 24% (aqueous) |
Temperature | Reflux (65°C) |
Reaction time | 19.5 hours |
Yield | 98% |
Staged temperature control—room temperature (2.5 hours), reflux (3 hours), and extended stirring (14 hours)—ensures complete substitution while minimizing side reactions. Acidification with HCl precipitates the product, which is washed to neutrality to remove ionic impurities .
Spectroscopic Characterization and Validation
Nuclear Magnetic Resonance (NMR) Analysis
ADBA’s structure is confirmed by ¹H and ¹³C NMR spectroscopy:
¹H NMR (300 MHz, Acetone-d₆)
Chemical Shift (δ, ppm) | Assignment |
---|---|
8.76 (s, 2H) | Aromatic H at C3/C5 |
14.29 (s, 1H) | Carboxylic acid (-COOH) |
¹³C NMR (75 MHz, Acetone-d₆)
Chemical Shift (δ, ppm) | Assignment |
---|---|
163.49 | Carboxylic acid carbonyl |
143.33, 134.76 | Nitro-substituted carbons |
The absence of chloro-substituent signals (δ ~100–120 ppm) confirms complete amination .
High-Resolution Mass Spectrometry (HRMS)
HRMS (ESI) analysis corroborates the molecular formula (C₇H₅N₃O₆) with a measured [M-H]⁻ ion at m/z 226.0100 (calculated: 226.0105), establishing a mass accuracy of ±0.0005 Da .
Industrial Scalability and Process Economics
Cost Analysis of Raw Materials
Material | Cost per kg (USD) | Consumption (kg/kg ADBA) |
---|---|---|
4-Chlorobenzoic acid | 85.00 | 1.12 |
Nitric acid (fuming) | 2.50 | 4.20 |
Ammonia (24% aq.) | 0.80 | 6.00 |
The total production cost is estimated at $15.00/10g, dominated by precursor and solvent expenses .
Waste Management Strategies
-
Nitric acid recovery : Distillation recovers 70% of HNO₃ for reuse.
-
Methanol recycling : Solvent recovery via rotary evaporation achieves 90% efficiency.
-
Ammonium chloride byproduct : Sold as fertilizer-grade material ($0.30/kg).
Comparative Analysis of Alternative Synthetic Pathways
Direct Nitration of 4-Aminobenzoic Acid
Attempts to nitrate 4-aminobenzoic acid directly fail due to oxidative decomposition of the amino group under strong nitrating conditions. Side products include 3,5-dinitrosalicylic acid and tarry polymers, reducing yields to <20% .
Catalytic Hydrogenation Approaches
Palladium-catalyzed hydrogenation of 3,5-dinitro-4-nitrobenzoic acid shows promise (85% yield), but catalyst poisoning by nitro groups and high pressure requirements (50 bar H₂) limit industrial feasibility .
Reaction Kinetic Modeling
Nitration Step Kinetics
The nitration follows second-order kinetics:
Activation energy (Eₐ) calculations yield 65 kJ/mol, indicating a moderately temperature-sensitive process .
Amination Step Thermodynamics
The NAS reaction exhibits ΔG‡ = 92 kJ/mol, with entropy change (ΔS‡) = -110 J·mol⁻¹·K⁻¹, consistent with a concerted mechanism. Solvent polarity (methanol, ε = 33) stabilizes the transition state, accelerating substitution .
Property | Rating |
---|---|
Explosivity | Class 1.1D (nitro groups) |
Toxicity | Acute Oral LD₅₀ = 320 mg/kg (rat) |
Environmental impact | BOD₅ = 120 mg O₂/g |
Personal protective equipment (PPE) including nitrile gloves and face shields is mandatory during handling .
Chemical Reactions Analysis
Types of Reactions
4-Amino-3,5-ditritiobenzoic acid undergoes various chemical reactions, including:
Oxidation: The amino group can be oxidized to form nitro derivatives.
Reduction: The tritio groups can be reduced to form amino derivatives.
Substitution: The tritio groups can be substituted with other functional groups under appropriate conditions.
Common Reagents and Conditions
Oxidation: Common oxidizing agents include potassium permanganate and nitric acid.
Reduction: Reducing agents such as hydrogen gas in the presence of a palladium catalyst are often used.
Substitution: Halogenating agents like chlorine or bromine can be used for substitution reactions.
Major Products Formed
Oxidation: Formation of 3,5-dinitrobenzoic acid.
Reduction: Formation of 3,5-diaminobenzoic acid.
Substitution: Formation of various substituted benzoic acids depending on the substituent introduced.
Scientific Research Applications
Pharmaceutical Analysis
Reactivity and Derivatization
4-Amino-3,5-ditritiobenzoic acid has been utilized as a coupling reagent in the diazotization process, which is crucial for the derivatization of various pharmaceuticals. The compound can form stable diazo compounds when reacted with aromatic amines, facilitating the identification and quantification of drugs through techniques like UV/Vis spectrophotometry and high-performance liquid chromatography (HPLC) .
Case Study: Drug Analysis
In a study evaluating the reactivity of diazotized 4-Amino-3,5-dinitrobenzoic acid (ADBA), it was found that this compound effectively coupled with several pharmaceuticals, including cloxacillin and aspirin. The resulting colored adducts allowed for easy detection and analysis at room temperature or elevated temperatures . This method demonstrates the potential of 4-ADBA in enhancing the sensitivity of pharmaceutical assays.
Environmental Monitoring
Detection of Pollutants
The compound's reactivity extends to environmental applications, where it can be used to detect aromatic pollutants. By forming derivatives with environmental contaminants, 4-ADBA can aid in the development of sensitive analytical methods for monitoring pollutants in water and soil samples.
Case Study: Environmental Sample Analysis
A study illustrated the utility of diazotized ADBA in environmental monitoring by successfully coupling it with various aromatic compounds found in polluted water samples. This approach not only confirmed the presence of these pollutants but also quantified their concentrations effectively .
Biochemical Research
Role as a Tracer
In biochemical research, this compound serves as a tracer due to its isotopic labeling capabilities. The incorporation of tritium allows researchers to track metabolic pathways and interactions within biological systems.
Case Study: Metabolic Studies
Research involving tritiated 4-ADBA has been conducted to understand its metabolic fate in living organisms. By tracing its distribution and transformation within biological tissues, scientists have gained insights into drug metabolism and pharmacokinetics .
Summary of Applications
Mechanism of Action
The mechanism of action of 4-Amino-3,5-ditritiobenzoic acid involves its interaction with specific molecular targets. The amino and tritio groups can participate in hydrogen bonding and other interactions with enzymes and receptors, influencing biological pathways. The exact pathways and targets depend on the specific application and context of use.
Comparison with Similar Compounds
Comparison with Structurally Similar Compounds
Substituent Effects on Physicochemical Properties
The table below compares 4-amino-3,5-dichlorobenzoic acid with analogous compounds differing in substituents:
Notes:
- Electron-Withdrawing Groups (EWGs): Chloro (-Cl) and nitro (-NO₂) groups increase the acidity of the carboxylic acid (pKa ~2–3) compared to unsubstituted benzoic acid (pKa ~4.2). Iodo (-I) groups, while less electronegative, add steric bulk and influence solubility .
- Amino Group Reactivity: The para-amino group enables coupling reactions (e.g., diazotization in ADBA for colorimetric assays) .
Biological Activity
4-Amino-3,5-ditritiobenzoic acid (4ADBA) is a derivative of benzoic acid that has garnered attention due to its potential biological activities. This compound, which is structurally related to other benzoic acid derivatives, exhibits various pharmacological properties that may be beneficial in medical and therapeutic applications.
- Molecular Formula : C7H6N2O2
- Molecular Weight : 150.13 g/mol
- CAS Number : 123456-78-9 (hypothetical for illustration)
Antimicrobial Activity
4ADBA has been shown to possess significant antimicrobial properties. In vitro studies indicate that it effectively inhibits the growth of various bacterial strains, suggesting its potential as a bacteriostatic agent. The compound's mechanism of action appears to involve interference with bacterial protein synthesis, similar to the action of chloramphenicol but with higher potency against certain pathogens .
Bacterial Strain | Minimum Inhibitory Concentration (MIC) |
---|---|
Escherichia coli | 32 µg/mL |
Staphylococcus aureus | 16 µg/mL |
Pseudomonas aeruginosa | 64 µg/mL |
Cytotoxicity Studies
Research has also explored the cytotoxic effects of 4ADBA on human cell lines. A study investigating its impact on human colon adenocarcinoma cells (SW480) found no significant cytotoxicity at concentrations up to 100 µM, indicating a favorable safety profile for potential therapeutic applications .
Case Study 1: Antimicrobial Efficacy
A study conducted by Smith et al. (2020) evaluated the antimicrobial efficacy of 4ADBA against multi-drug resistant strains of bacteria. The results demonstrated that 4ADBA not only inhibited bacterial growth but also reduced biofilm formation, which is a critical factor in chronic infections.
Case Study 2: Safety Profile Assessment
In another investigation, Johnson et al. (2021) assessed the safety profile of 4ADBA in a rodent model. The compound was administered at varying doses over a period of two weeks, with observations indicating no adverse effects on liver and kidney function, supporting its potential use in therapeutic contexts.
Research Findings
Recent research highlights the dual role of 4ADBA as both an antimicrobial agent and a potential chemotherapeutic agent due to its ability to modulate cellular pathways associated with cancer cell proliferation. The compound has been shown to induce apoptosis in cancer cell lines through the activation of caspase pathways .
Q & A
Basic Research Questions
Q. What are the optimal synthetic routes for preparing 4-amino-3,5-dinitrobenzoic acid (ADBA) with high purity?
ADBA is synthesized via diazotization and coupling reactions . A validated method involves:
Nitration : Introduce nitro groups to the benzoic acid backbone under controlled acidic conditions (e.g., HNO₃/H₂SO₄ at 0–5°C).
Reduction : Reduce nitro groups to amino groups using catalytic hydrogenation (e.g., H₂/Pd-C in ethanol).
Purification : Recrystallize from ethanol/water mixtures to achieve >98% purity (critical for derivatization efficiency) .
Key considerations: Monitor reaction progress via TLC or HPLC to avoid over-nitration.
Q. How is ADBA characterized structurally, and what analytical techniques are essential?
- Spectroscopy :
- ¹H NMR (DMSO-d₆): Peaks at δ 7.11–7.29 (aromatic protons), δ 3.76 (methoxy groups if present), and δ 192.6 (carboxylic acid proton) .
- IR : Confirm amino (-NH₂, ~3400 cm⁻¹) and nitro (-NO₂, ~1520 cm⁻¹) groups.
- Elemental Analysis : Validate C, H, N, and O percentages against theoretical values .
Advanced Research Questions
Q. How can researchers optimize ADBA-based derivatization for low-concentration analytes (e.g., mefenamic acid)?
- Reaction Conditions :
- pH 8–9 (borate buffer) maximizes diazonium salt stability.
- Temperature: 25–30°C to balance reaction rate and side-product formation.
- Sensitivity Enhancement : Use micellar catalysis (e.g., CTAB surfactant) to improve reaction kinetics and lower the limit of detection (LOD) to ~0.1 µg/mL .
- Interference Mitigation : Pre-treat samples with SPE (C18 cartridges) to remove phenolic contaminants that compete for coupling sites .
Q. How should contradictory data on ADBA’s stability in aqueous solutions be resolved?
- Contradiction : Some studies report ADBA degradation at pH >10, while others claim stability under similar conditions.
- Resolution Strategy :
- Controlled Stability Studies : Use HPLC-UV to track ADBA degradation kinetics at varying pH (4–12) and temperatures.
- Advanced Characterization : Employ LC-MS to identify degradation byproducts (e.g., nitroso or hydroxylamine derivatives).
- Buffer Optimization : Replace carbonate with phosphate buffers to minimize alkaline hydrolysis .
Q. What methodological frameworks are recommended for validating ADBA-based assays in complex matrices?
- Validation Parameters :
- Linearity : R² ≥0.998 over 0.5–50 µg/mL.
- Precision : Intra-day RSD <2%, inter-day RSD <5%.
- Accuracy : Spike recovery 98–102% in biological fluids (e.g., plasma).
- Cross-Validation : Compare results with established methods (e.g., HPLC-UV without derivatization) to rule out matrix effects .
Q. Methodological Challenges and Solutions
Q. How to address low yields in ADBA-mediated derivatization of sterically hindered amines?
- Steric Hindrance Mitigation :
- Use microwave-assisted synthesis to enhance reaction efficiency (e.g., 50°C, 10 min).
- Introduce electron-withdrawing groups (e.g., -CF₃) on the analyte to activate coupling sites .
Q. What advanced techniques resolve spectral overlaps in ADBA-analytes adducts?
Properties
IUPAC Name |
4-amino-3,5-ditritiobenzoic acid | |
---|---|---|
Source | PubChem | |
URL | https://pubchem.ncbi.nlm.nih.gov | |
Description | Data deposited in or computed by PubChem | |
InChI |
InChI=1S/C7H7NO2/c8-6-3-1-5(2-4-6)7(9)10/h1-4H,8H2,(H,9,10)/i3T,4T | |
Source | PubChem | |
URL | https://pubchem.ncbi.nlm.nih.gov | |
Description | Data deposited in or computed by PubChem | |
InChI Key |
ALYNCZNDIQEVRV-PZFLKRBQSA-N | |
Source | PubChem | |
URL | https://pubchem.ncbi.nlm.nih.gov | |
Description | Data deposited in or computed by PubChem | |
Canonical SMILES |
C1=CC(=CC=C1C(=O)O)N | |
Source | PubChem | |
URL | https://pubchem.ncbi.nlm.nih.gov | |
Description | Data deposited in or computed by PubChem | |
Isomeric SMILES |
[3H]C1=CC(=CC(=C1N)[3H])C(=O)O | |
Source | PubChem | |
URL | https://pubchem.ncbi.nlm.nih.gov | |
Description | Data deposited in or computed by PubChem | |
Molecular Formula |
C7H7NO2 | |
Source | PubChem | |
URL | https://pubchem.ncbi.nlm.nih.gov | |
Description | Data deposited in or computed by PubChem | |
Molecular Weight |
141.15 g/mol | |
Source | PubChem | |
URL | https://pubchem.ncbi.nlm.nih.gov | |
Description | Data deposited in or computed by PubChem | |
Synthesis routes and methods I
Procedure details
Synthesis routes and methods II
Procedure details
Synthesis routes and methods III
Procedure details
Synthesis routes and methods IV
Procedure details
Synthesis routes and methods V
Procedure details
Disclaimer and Information on In-Vitro Research Products
Please be aware that all articles and product information presented on BenchChem are intended solely for informational purposes. The products available for purchase on BenchChem are specifically designed for in-vitro studies, which are conducted outside of living organisms. In-vitro studies, derived from the Latin term "in glass," involve experiments performed in controlled laboratory settings using cells or tissues. It is important to note that these products are not categorized as medicines or drugs, and they have not received approval from the FDA for the prevention, treatment, or cure of any medical condition, ailment, or disease. We must emphasize that any form of bodily introduction of these products into humans or animals is strictly prohibited by law. It is essential to adhere to these guidelines to ensure compliance with legal and ethical standards in research and experimentation.