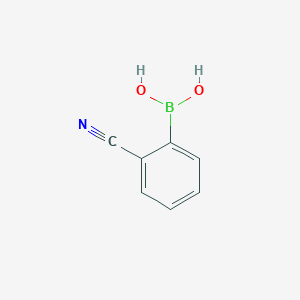
2-Cyanophenylboronic acid
Overview
Description
2-Cyanophenylboronic acid is an off-white to cream powder . It has the molecular formula C7H6BNO2 . It is used in the synthesis of pyridine inhibitors of TAK1 and also participates in the discovery and synthesis of 1,2,4-triazoles as novel allosteric valosine containing protein (VCP) inhibitors .
Synthesis Analysis
2-Cyanophenylboronic acid is employed in a rhodium-catalyzed [3+2] annulation with alkynes leading to substituted indenones . It is also useful in the synthesis of substitutes indenones or indanones . It is used in the synthesis of pyridine inhibitors of TAK1 .Molecular Structure Analysis
The molecular structure of 2-Cyanophenylboronic acid is characterized by the presence of a cyanophenyl group attached to a boronic acid group . The molecular weight is 146.94 Da .Chemical Reactions Analysis
2-Cyanophenylboronic acid is employed in a rhodium-catalyzed [3+2] annulation with alkynes leading to substituted indenones . It is also useful in the synthesis of substitutes indenones or indanones .Physical And Chemical Properties Analysis
2-Cyanophenylboronic acid has a density of 1.3±0.1 g/cm3, a boiling point of 376.4±44.0 °C at 760 mmHg, and a flash point of 181.4±28.4 °C . It has 3 hydrogen bond acceptors, 2 hydrogen bond donors, and 2 freely rotating bonds .Scientific Research Applications
- Details : The reaction involves the use of 2-cyanophenylboronic acid as a three-carbon component, which reacts with alkynes or alkenes to afford substituted indenones or indanones. Remarkably, even alkynoates can produce benzotropone, a valuable compound .
- Details : Researchers have employed 2-cyanophenylboronic acid to construct these important heterocyclic compounds. The resulting products have applications in medicinal chemistry, materials science, and organic synthesis .
- Details : Researchers have explored the use of 2-cyanophenylboronic acid in designing molecular receptors. These receptors can selectively bind to specific analytes, making them valuable for sensing and molecular recognition studies .
- Details : 2-cyanophenylboronic acid derivatives have been investigated as potential ligands for histamine-3 receptors. Understanding their binding interactions can aid drug discovery efforts targeting histamine receptors .
- Details : Researchers have explored the use of 2-cyanophenylboronic acid in designing functional materials, such as polymers, liquid crystals, and supramolecular assemblies. Its boronic acid moiety can participate in reversible interactions, making it useful for responsive materials .
- Details : 2-cyanophenylboronic acid serves as a versatile reagent in Suzuki-Miyaura cross-coupling reactions, allowing the introduction of the cyanophenyl group into various organic molecules. This strategy enables the synthesis of diverse compounds for drug discovery and materials science .
Rhodium-Catalyzed Annulation
Synthesis of Indenones and Indanones
Oligo-Boronic Acid Receptors
Histamine-3 Receptor Ligands
Materials Chemistry
Organic Synthesis and Cross-Coupling Reactions
properties
IUPAC Name |
(2-cyanophenyl)boronic acid | |
---|---|---|
Source | PubChem | |
URL | https://pubchem.ncbi.nlm.nih.gov | |
Description | Data deposited in or computed by PubChem | |
InChI |
InChI=1S/C7H6BNO2/c9-5-6-3-1-2-4-7(6)8(10)11/h1-4,10-11H | |
Source | PubChem | |
URL | https://pubchem.ncbi.nlm.nih.gov | |
Description | Data deposited in or computed by PubChem | |
InChI Key |
NPLZNDDFVCGRAG-UHFFFAOYSA-N | |
Source | PubChem | |
URL | https://pubchem.ncbi.nlm.nih.gov | |
Description | Data deposited in or computed by PubChem | |
Canonical SMILES |
B(C1=CC=CC=C1C#N)(O)O | |
Source | PubChem | |
URL | https://pubchem.ncbi.nlm.nih.gov | |
Description | Data deposited in or computed by PubChem | |
Molecular Formula |
C7H6BNO2 | |
Source | PubChem | |
URL | https://pubchem.ncbi.nlm.nih.gov | |
Description | Data deposited in or computed by PubChem | |
DSSTOX Substance ID |
DTXSID00370391 | |
Record name | 2-Cyanophenylboronic acid | |
Source | EPA DSSTox | |
URL | https://comptox.epa.gov/dashboard/DTXSID00370391 | |
Description | DSSTox provides a high quality public chemistry resource for supporting improved predictive toxicology. | |
Molecular Weight |
146.94 g/mol | |
Source | PubChem | |
URL | https://pubchem.ncbi.nlm.nih.gov | |
Description | Data deposited in or computed by PubChem | |
Product Name |
2-Cyanophenylboronic acid | |
CAS RN |
138642-62-3 | |
Record name | 2-Cyanophenylboronic acid | |
Source | CAS Common Chemistry | |
URL | https://commonchemistry.cas.org/detail?cas_rn=138642-62-3 | |
Description | CAS Common Chemistry is an open community resource for accessing chemical information. Nearly 500,000 chemical substances from CAS REGISTRY cover areas of community interest, including common and frequently regulated chemicals, and those relevant to high school and undergraduate chemistry classes. This chemical information, curated by our expert scientists, is provided in alignment with our mission as a division of the American Chemical Society. | |
Explanation | The data from CAS Common Chemistry is provided under a CC-BY-NC 4.0 license, unless otherwise stated. | |
Record name | 2-Cyanophenylboronic acid | |
Source | EPA DSSTox | |
URL | https://comptox.epa.gov/dashboard/DTXSID00370391 | |
Description | DSSTox provides a high quality public chemistry resource for supporting improved predictive toxicology. | |
Synthesis routes and methods I
Procedure details
Synthesis routes and methods II
Procedure details
Synthesis routes and methods III
Procedure details
Retrosynthesis Analysis
AI-Powered Synthesis Planning: Our tool employs the Template_relevance Pistachio, Template_relevance Bkms_metabolic, Template_relevance Pistachio_ringbreaker, Template_relevance Reaxys, Template_relevance Reaxys_biocatalysis model, leveraging a vast database of chemical reactions to predict feasible synthetic routes.
One-Step Synthesis Focus: Specifically designed for one-step synthesis, it provides concise and direct routes for your target compounds, streamlining the synthesis process.
Accurate Predictions: Utilizing the extensive PISTACHIO, BKMS_METABOLIC, PISTACHIO_RINGBREAKER, REAXYS, REAXYS_BIOCATALYSIS database, our tool offers high-accuracy predictions, reflecting the latest in chemical research and data.
Strategy Settings
Precursor scoring | Relevance Heuristic |
---|---|
Min. plausibility | 0.01 |
Model | Template_relevance |
Template Set | Pistachio/Bkms_metabolic/Pistachio_ringbreaker/Reaxys/Reaxys_biocatalysis |
Top-N result to add to graph | 6 |
Feasible Synthetic Routes
Q & A
Q1: What makes 2-cyanophenylboronic acid a unique reagent in organic synthesis?
A1: 2-Cyanophenylboronic acid distinguishes itself by acting as a three-carbon building block in rhodium-catalyzed annulations with alkynes and strained alkenes [, ]. This versatility allows for the construction of valuable cyclic structures like indenones and indanones. Notably, reactions with alkynoates even yield benzotropone, demonstrating its potential in forming complex ring systems [].
Q2: Could you elaborate on the mechanism by which 2-cyanophenylboronic acid participates in these reactions?
A2: Research suggests that the reaction proceeds through the formation of an organorhodium(I) intermediate from 2-cyanophenylboronic acid under rhodium catalysis []. This intermediate then undergoes intramolecular nucleophilic addition to the cyano group, ultimately leading to the formation of the cyclic product. This crucial step highlights the dual role of the cyano group, acting as both a directing group for the metal catalyst and a reactive site for cyclization [].
Disclaimer and Information on In-Vitro Research Products
Please be aware that all articles and product information presented on BenchChem are intended solely for informational purposes. The products available for purchase on BenchChem are specifically designed for in-vitro studies, which are conducted outside of living organisms. In-vitro studies, derived from the Latin term "in glass," involve experiments performed in controlled laboratory settings using cells or tissues. It is important to note that these products are not categorized as medicines or drugs, and they have not received approval from the FDA for the prevention, treatment, or cure of any medical condition, ailment, or disease. We must emphasize that any form of bodily introduction of these products into humans or animals is strictly prohibited by law. It is essential to adhere to these guidelines to ensure compliance with legal and ethical standards in research and experimentation.