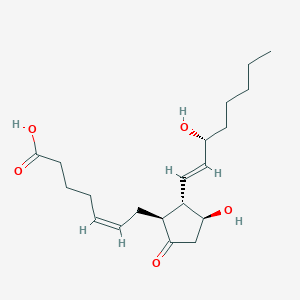
ent-PGE2
Overview
Description
ent-Prostaglandin E2 is a stereoisomer of Prostaglandin E2, a naturally occurring prostaglandin with significant biological activities. Prostaglandins are lipid compounds derived from fatty acids and have diverse roles in the human body, including the regulation of inflammation, blood flow, and the formation of blood clots. ent-Prostaglandin E2, specifically, is known for its involvement in various physiological processes and potential therapeutic applications.
Mechanism of Action
Target of Action
The primary targets of ent-PGE2 are the prostaglandin E2 receptors (EP1-4) . These receptors are G protein-coupled receptors with distinct signaling properties . EP2 receptor, in particular, has been shown to mediate the effects of PGE2 on macrophages .
Mode of Action
This compound interacts with its targets, the EP receptors, to induce various downstream effects. For instance, PGE2-EP2 signaling activates PKB, leading to the activation of glycogen synthase and thus glycogen synthesis . Moreover, PGE2 can influence blood pressure both positively and negatively. Centrally administered PGE2 induces hypertension, whereas systemic administration of PGE2 produces a hypotensive effect .
Biochemical Pathways
PGE2 is derived from arachidonic acid (AA) via the actions of the enzyme cytosolic phospholipase A2 (cPLA2), which generates AA. Free AA can then be metabolized via the enzymatic activity of cyclooxygenase 1 or 2 (COX-1 or COX-2) and PGE synthase 1 . PGE2 seems to act on monocyte-derived macrophages (MDMs) in an autocrine fashion to alter metabolism .
Result of Action
The action of this compound leads to various molecular and cellular effects. For instance, it has been shown to increase neurite length and the proportion of neurites that form axonal loops in differentiating NE4C cells . Additionally, PGE2 has been found to reduce oxidative phosphorylation and glycolysis in MDMs , and to promote macrophage recruitment and neovascularization in murine wet-type AMD models .
Action Environment
The action of this compound can be influenced by various environmental factors. For example, abnormal levels of PGE2 due to environmental insults during prenatal development have been linked to brain pathologies . Furthermore, the level of PGE2 expression was found to be high in laser-induced CNV lesions, suggesting that the local environment can influence the action and efficacy of PGE2 .
Biochemical Analysis
Biochemical Properties
ent-Prostaglandin E2 plays a significant role in biochemical reactions. It interacts with various enzymes, proteins, and other biomolecules. For instance, it is involved in the biosynthesis of PGE2, a crucial biochemical signal mediating immune and reproductive physiology . The biosynthesis of ent-Prostaglandin E2 begins with the hydrolysis of arachidonic acid from cellular phospholipids by a phospholipase A2 .
Cellular Effects
ent-Prostaglandin E2 has profound effects on various types of cells and cellular processes. It influences cell function, including impacting cell signaling pathways, gene expression, and cellular metabolism . For example, it plays a relevant role in the ovulatory cascade, including meiotic maturation, cumulus expansion, and follicle rupture .
Molecular Mechanism
The molecular mechanism of ent-Prostaglandin E2 involves its binding interactions with biomolecules, enzyme inhibition or activation, and changes in gene expression . It mediates the biosynthesis of PGE2 through its cognate protein .
Metabolic Pathways
ent-Prostaglandin E2 is involved in several metabolic pathways. It interacts with enzymes or cofactors, and it can affect metabolic flux or metabolite levels .
Preparation Methods
Synthetic Routes and Reaction Conditions: The synthesis of ent-Prostaglandin E2 typically involves the Corey synthesis method, which is widely used for the production of prostaglandins. The key intermediate in this synthesis is the Corey lactone, from which the omega and alpha side chains of prostaglandins can be constructed. The process involves several steps, including the formation of a lactone ring, followed by the addition of side chains through various chemical reactions .
Industrial Production Methods: Industrial production of ent-Prostaglandin E2 involves large-scale synthesis using similar methods as described above. The process is optimized for higher yields and purity, often involving chiral high-performance liquid chromatography (HPLC) for the separation of enantiomers . The use of advanced techniques ensures the production of high-quality ent-Prostaglandin E2 for research and therapeutic purposes.
Chemical Reactions Analysis
Types of Reactions: ent-Prostaglandin E2 undergoes various chemical reactions, including:
Oxidation: Conversion of hydroxyl groups to ketones or aldehydes.
Reduction: Reduction of ketones to hydroxyl groups.
Substitution: Replacement of functional groups with other substituents.
Common Reagents and Conditions:
Oxidation: Common oxidizing agents include potassium permanganate and chromium trioxide.
Reduction: Reducing agents such as sodium borohydride and lithium aluminum hydride are used.
Substitution: Conditions vary depending on the substituent being introduced, but often involve the use of catalysts and specific solvents.
Major Products: The major products formed from these reactions depend on the specific conditions and reagents used. For example, oxidation of ent-Prostaglandin E2 can lead to the formation of ketones, while reduction can yield hydroxyl derivatives.
Scientific Research Applications
ent-Prostaglandin E2 has a wide range of scientific research applications, including:
Chemistry: Used as a standard for studying the stereochemistry and reactivity of prostaglandins.
Biology: Investigated for its role in cellular signaling and regulation of physiological processes.
Medicine: Explored for its potential therapeutic applications in treating inflammation, pain, and other conditions.
Industry: Utilized in the development of pharmaceuticals and as a reference compound in analytical methods
Comparison with Similar Compounds
Prostaglandin E2: The naturally occurring form with similar biological activities.
Prostaglandin F2α: Another prostaglandin with distinct roles in smooth muscle contraction and reproductive processes.
Prostaglandin D2: Involved in allergic reactions and sleep regulation.
Uniqueness: ent-Prostaglandin E2 is unique due to its specific stereochemistry, which can result in different biological activities compared to its naturally occurring counterpart. This makes it valuable for studying the effects of stereochemistry on prostaglandin function and for developing targeted therapeutic agents .
Properties
IUPAC Name |
(Z)-7-[(1S,2S,3S)-3-hydroxy-2-[(E,3R)-3-hydroxyoct-1-enyl]-5-oxocyclopentyl]hept-5-enoic acid | |
---|---|---|
Source | PubChem | |
URL | https://pubchem.ncbi.nlm.nih.gov | |
Description | Data deposited in or computed by PubChem | |
InChI |
InChI=1S/C20H32O5/c1-2-3-6-9-15(21)12-13-17-16(18(22)14-19(17)23)10-7-4-5-8-11-20(24)25/h4,7,12-13,15-17,19,21,23H,2-3,5-6,8-11,14H2,1H3,(H,24,25)/b7-4-,13-12+/t15-,16+,17+,19+/m1/s1 | |
Source | PubChem | |
URL | https://pubchem.ncbi.nlm.nih.gov | |
Description | Data deposited in or computed by PubChem | |
InChI Key |
XEYBRNLFEZDVAW-OBUVHCMGSA-N | |
Source | PubChem | |
URL | https://pubchem.ncbi.nlm.nih.gov | |
Description | Data deposited in or computed by PubChem | |
Canonical SMILES |
CCCCCC(C=CC1C(CC(=O)C1CC=CCCCC(=O)O)O)O | |
Source | PubChem | |
URL | https://pubchem.ncbi.nlm.nih.gov | |
Description | Data deposited in or computed by PubChem | |
Isomeric SMILES |
CCCCC[C@H](/C=C/[C@@H]1[C@H](CC(=O)[C@H]1C/C=C\CCCC(=O)O)O)O | |
Source | PubChem | |
URL | https://pubchem.ncbi.nlm.nih.gov | |
Description | Data deposited in or computed by PubChem | |
Molecular Formula |
C20H32O5 | |
Source | PubChem | |
URL | https://pubchem.ncbi.nlm.nih.gov | |
Description | Data deposited in or computed by PubChem | |
Molecular Weight |
352.5 g/mol | |
Source | PubChem | |
URL | https://pubchem.ncbi.nlm.nih.gov | |
Description | Data deposited in or computed by PubChem | |
Retrosynthesis Analysis
AI-Powered Synthesis Planning: Our tool employs the Template_relevance Pistachio, Template_relevance Bkms_metabolic, Template_relevance Pistachio_ringbreaker, Template_relevance Reaxys, Template_relevance Reaxys_biocatalysis model, leveraging a vast database of chemical reactions to predict feasible synthetic routes.
One-Step Synthesis Focus: Specifically designed for one-step synthesis, it provides concise and direct routes for your target compounds, streamlining the synthesis process.
Accurate Predictions: Utilizing the extensive PISTACHIO, BKMS_METABOLIC, PISTACHIO_RINGBREAKER, REAXYS, REAXYS_BIOCATALYSIS database, our tool offers high-accuracy predictions, reflecting the latest in chemical research and data.
Strategy Settings
Precursor scoring | Relevance Heuristic |
---|---|
Min. plausibility | 0.01 |
Model | Template_relevance |
Template Set | Pistachio/Bkms_metabolic/Pistachio_ringbreaker/Reaxys/Reaxys_biocatalysis |
Top-N result to add to graph | 6 |
Feasible Synthetic Routes
Q1: What is the significance of developing a chiral HPLC method for separating ent-Prostaglandin E2 enantiomers?
A1: [] Chiral HPLC separation is crucial for obtaining enantiomerically pure ent-Prostaglandin E2 ((+)-2), which is essential for several reasons. Firstly, it allows for the verification of enantiopurity in synthetically produced Prostaglandin E2. This is important because different enantiomers of a molecule can have different biological activities. Secondly, having pure ent-Prostaglandin E2 as an analytical standard enables researchers to study the oxidation processes of Prostaglandin E2 in vivo and potentially understand its metabolic pathways. []
Q2: How does the luteolytic potential of ent-Prostaglandin E2 compare to other prostaglandin analogues?
A2: While the provided abstracts don't directly compare the luteolytic activity of ent-Prostaglandin E2 to other analogues, they do highlight the effects of similar compounds. Notably, PGF2alpha-1,15-lactone, 11alpha(15S)-17-phenyl-18,19,20-trinor-ent-Prostaglandin E2 methyl ester, and 17-phenyl-18,19,20-trinor-PGF2alpha all demonstrated luteolytic potential in chacma baboons. [] Further research is needed to directly compare the potency and efficacy of ent-Prostaglandin E2 in this context.
Disclaimer and Information on In-Vitro Research Products
Please be aware that all articles and product information presented on BenchChem are intended solely for informational purposes. The products available for purchase on BenchChem are specifically designed for in-vitro studies, which are conducted outside of living organisms. In-vitro studies, derived from the Latin term "in glass," involve experiments performed in controlled laboratory settings using cells or tissues. It is important to note that these products are not categorized as medicines or drugs, and they have not received approval from the FDA for the prevention, treatment, or cure of any medical condition, ailment, or disease. We must emphasize that any form of bodily introduction of these products into humans or animals is strictly prohibited by law. It is essential to adhere to these guidelines to ensure compliance with legal and ethical standards in research and experimentation.