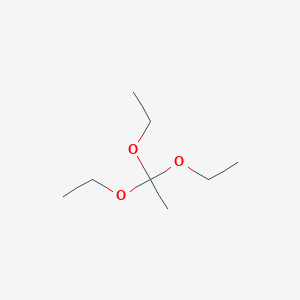
Triethyl orthoacetate
Overview
Description
Triethyl orthoacetate is an organic compound with the formula CH3C(OC2H5)3 . It is the ethyl orthoester of acetic acid and is a colorless oily liquid . It is used in organic synthesis for acetylation and also in the Johnson-Claisen rearrangement .
Synthesis Analysis
Ortho esters can be prepared by the Pinner reaction, in which nitriles react with alcohols in the presence of one equivalent of hydrogen chloride . The reaction proceeds by the formation of imido ester hydrochloride .Molecular Structure Analysis
The molecular formula of Triethyl orthoacetate is C8H18O3 . Its average mass is 162.227 Da and its monoisotopic mass is 162.125595 Da .Chemical Reactions Analysis
Triethyl orthoacetate is used in organic synthesis for acetylation . It is also used in the Johnson-Claisen rearrangement . The Johnson–Claisen rearrangement is the reaction of an allylic alcohol with an ortho ester containing a deprotonatable alpha carbon (e.g. triethyl orthoacetate) to give a γ,δ-unsaturated ester .Physical And Chemical Properties Analysis
Triethyl orthoacetate has a boiling point of 142 °C and a density of 0.885 g/mL . Its refractive index is 1.396 .Scientific Research Applications
Synthesis of Ordered Mesoporous Carbons
Triethyl orthoacetate can be used in the synthesis of ordered mesoporous carbons . These materials have applications in catalysis, electrochemistry, and hydrogen storage due to their unique structural properties .
Synthesis of Esters
Triethyl orthoacetate is used in the synthesis of esters of phosphonic acids, carboxylic acids, and phosphinic acids . These esters have a wide range of applications in pharmaceuticals and other chemical industries .
Johnson–Claisen Rearrangement Reaction
This compound is used in the Johnson–Claisen rearrangement reaction to produce Ethyl alk-4-enoates . This reaction is a powerful tool in organic synthesis for the formation of carbon-carbon bonds .
Synthesis of Benzoxazoles, Benzimidazoles, and Oxazolo[4,5-b]pyridines
Triethyl orthoacetate is used in the synthesis of benzoxazoles, benzimidazoles, and oxazolo[4,5-b]pyridines . These compounds have significant importance in medicinal chemistry due to their wide range of biological activities .
Triethyl orthoacetate is used in organic synthesis to introduce the acetate group into an alcohol . This process is crucial in the production of various pharmaceuticals and other organic compounds .
Water Scavenger
Triethyl orthoacetate is used as a water scavenger . It reacts with water to form acetic acid and ethanol, thereby removing water from reactions where it could interfere with the desired outcome .
Mechanism of Action
Target of Action
Triethyl orthoacetate, also known as 1,1,1-Triethoxyethane, is primarily used in organic synthesis . Its primary targets are various organic compounds that undergo acetylation . It is also used in the Johnson-Claisen rearrangement .
Mode of Action
Triethyl orthoacetate interacts with its targets through a process known as acetylation . This involves the transfer of an acetyl group from the orthoacetate to the target molecule . In the Johnson-Claisen rearrangement, it acts as a reagent to facilitate the rearrangement of allylic alcohols .
Biochemical Pathways
The primary biochemical pathway affected by Triethyl orthoacetate is the acetylation pathway . Acetylation is a critical process in organic chemistry, affecting the structure and function of molecules . Additionally, it plays a role in the Johnson-Claisen rearrangement, a reaction that results in the formation of γ,δ-unsaturated esters .
Pharmacokinetics
It’s worth noting that its physical properties, such as its boiling point (142°c) and density (0885 g/mL at 25°C), can influence its behavior in a chemical reaction .
Result of Action
The result of Triethyl orthoacetate’s action is the acetylation of target molecules, altering their structure and potentially their function . In the context of the Johnson-Claisen rearrangement, it helps facilitate the rearrangement of allylic alcohols to form γ,δ-unsaturated esters .
Action Environment
The action of Triethyl orthoacetate can be influenced by environmental factors. For instance, it is stable in alkaline conditions but decomposes in the presence of water and acidic media . It is also flammable and should be kept away from heat, hot surfaces, sparks, open flames, and other ignition sources . Its efficacy and stability can be affected by these and other environmental factors .
Safety and Hazards
Triethyl orthoacetate is a flammable liquid and vapor. It causes skin irritation and serious eye irritation . Precautionary measures include avoiding contact with skin and eyes, not breathing mist/vapors/spray, taking precautionary measures against static discharges, using spark-proof tools and explosion-proof equipment, and keeping away from open flames, hot surfaces, and sources of ignition .
Future Directions
Triethyl orthoacetate is a valuable and efficient substrate to perform various classes of two-component and multi-component organic reactions . The authors of a review on applications of alkyl orthoesters hope their assessment will help chemists to attain new approaches for utilizing alkyl orthoesters in various organic synthetic methods .
properties
IUPAC Name |
1,1,1-triethoxyethane | |
---|---|---|
Source | PubChem | |
URL | https://pubchem.ncbi.nlm.nih.gov | |
Description | Data deposited in or computed by PubChem | |
InChI |
InChI=1S/C8H18O3/c1-5-9-8(4,10-6-2)11-7-3/h5-7H2,1-4H3 | |
Source | PubChem | |
URL | https://pubchem.ncbi.nlm.nih.gov | |
Description | Data deposited in or computed by PubChem | |
InChI Key |
NDQXKKFRNOPRDW-UHFFFAOYSA-N | |
Source | PubChem | |
URL | https://pubchem.ncbi.nlm.nih.gov | |
Description | Data deposited in or computed by PubChem | |
Canonical SMILES |
CCOC(C)(OCC)OCC | |
Source | PubChem | |
URL | https://pubchem.ncbi.nlm.nih.gov | |
Description | Data deposited in or computed by PubChem | |
Molecular Formula |
C8H18O3 | |
Source | PubChem | |
URL | https://pubchem.ncbi.nlm.nih.gov | |
Description | Data deposited in or computed by PubChem | |
DSSTOX Substance ID |
DTXSID4058815 | |
Record name | Ethane, 1,1,1-triethoxy- | |
Source | EPA DSSTox | |
URL | https://comptox.epa.gov/dashboard/DTXSID4058815 | |
Description | DSSTox provides a high quality public chemistry resource for supporting improved predictive toxicology. | |
Molecular Weight |
162.23 g/mol | |
Source | PubChem | |
URL | https://pubchem.ncbi.nlm.nih.gov | |
Description | Data deposited in or computed by PubChem | |
Physical Description |
Colorless liquid; [Aldrich MSDS] | |
Record name | 1,1,1-Triethoxyethane | |
Source | Haz-Map, Information on Hazardous Chemicals and Occupational Diseases | |
URL | https://haz-map.com/Agents/9803 | |
Description | Haz-Map® is an occupational health database designed for health and safety professionals and for consumers seeking information about the adverse effects of workplace exposures to chemical and biological agents. | |
Explanation | Copyright (c) 2022 Haz-Map(R). All rights reserved. Unless otherwise indicated, all materials from Haz-Map are copyrighted by Haz-Map(R). No part of these materials, either text or image may be used for any purpose other than for personal use. Therefore, reproduction, modification, storage in a retrieval system or retransmission, in any form or by any means, electronic, mechanical or otherwise, for reasons other than personal use, is strictly prohibited without prior written permission. | |
Product Name |
Triethyl orthoacetate | |
CAS RN |
78-39-7 | |
Record name | Triethyl orthoacetate | |
Source | CAS Common Chemistry | |
URL | https://commonchemistry.cas.org/detail?cas_rn=78-39-7 | |
Description | CAS Common Chemistry is an open community resource for accessing chemical information. Nearly 500,000 chemical substances from CAS REGISTRY cover areas of community interest, including common and frequently regulated chemicals, and those relevant to high school and undergraduate chemistry classes. This chemical information, curated by our expert scientists, is provided in alignment with our mission as a division of the American Chemical Society. | |
Explanation | The data from CAS Common Chemistry is provided under a CC-BY-NC 4.0 license, unless otherwise stated. | |
Record name | 1,1,1-Triethoxyethane | |
Source | ChemIDplus | |
URL | https://pubchem.ncbi.nlm.nih.gov/substance/?source=chemidplus&sourceid=0000078397 | |
Description | ChemIDplus is a free, web search system that provides access to the structure and nomenclature authority files used for the identification of chemical substances cited in National Library of Medicine (NLM) databases, including the TOXNET system. | |
Record name | Triethyl orthoacetate | |
Source | DTP/NCI | |
URL | https://dtp.cancer.gov/dtpstandard/servlet/dwindex?searchtype=NSC&outputformat=html&searchlist=5596 | |
Description | The NCI Development Therapeutics Program (DTP) provides services and resources to the academic and private-sector research communities worldwide to facilitate the discovery and development of new cancer therapeutic agents. | |
Explanation | Unless otherwise indicated, all text within NCI products is free of copyright and may be reused without our permission. Credit the National Cancer Institute as the source. | |
Record name | Ethane, 1,1,1-triethoxy- | |
Source | EPA Chemicals under the TSCA | |
URL | https://www.epa.gov/chemicals-under-tsca | |
Description | EPA Chemicals under the Toxic Substances Control Act (TSCA) collection contains information on chemicals and their regulations under TSCA, including non-confidential content from the TSCA Chemical Substance Inventory and Chemical Data Reporting. | |
Record name | Ethane, 1,1,1-triethoxy- | |
Source | EPA DSSTox | |
URL | https://comptox.epa.gov/dashboard/DTXSID4058815 | |
Description | DSSTox provides a high quality public chemistry resource for supporting improved predictive toxicology. | |
Record name | Triethyl orthoacetate | |
Source | European Chemicals Agency (ECHA) | |
URL | https://echa.europa.eu/substance-information/-/substanceinfo/100.001.012 | |
Description | The European Chemicals Agency (ECHA) is an agency of the European Union which is the driving force among regulatory authorities in implementing the EU's groundbreaking chemicals legislation for the benefit of human health and the environment as well as for innovation and competitiveness. | |
Explanation | Use of the information, documents and data from the ECHA website is subject to the terms and conditions of this Legal Notice, and subject to other binding limitations provided for under applicable law, the information, documents and data made available on the ECHA website may be reproduced, distributed and/or used, totally or in part, for non-commercial purposes provided that ECHA is acknowledged as the source: "Source: European Chemicals Agency, http://echa.europa.eu/". Such acknowledgement must be included in each copy of the material. ECHA permits and encourages organisations and individuals to create links to the ECHA website under the following cumulative conditions: Links can only be made to webpages that provide a link to the Legal Notice page. | |
Record name | 1,1,1-TRIETHOXYETHANE | |
Source | FDA Global Substance Registration System (GSRS) | |
URL | https://gsrs.ncats.nih.gov/ginas/app/beta/substances/48352MHC78 | |
Description | The FDA Global Substance Registration System (GSRS) enables the efficient and accurate exchange of information on what substances are in regulated products. Instead of relying on names, which vary across regulatory domains, countries, and regions, the GSRS knowledge base makes it possible for substances to be defined by standardized, scientific descriptions. | |
Explanation | Unless otherwise noted, the contents of the FDA website (www.fda.gov), both text and graphics, are not copyrighted. They are in the public domain and may be republished, reprinted and otherwise used freely by anyone without the need to obtain permission from FDA. Credit to the U.S. Food and Drug Administration as the source is appreciated but not required. | |
Synthesis routes and methods
Procedure details
Retrosynthesis Analysis
AI-Powered Synthesis Planning: Our tool employs the Template_relevance Pistachio, Template_relevance Bkms_metabolic, Template_relevance Pistachio_ringbreaker, Template_relevance Reaxys, Template_relevance Reaxys_biocatalysis model, leveraging a vast database of chemical reactions to predict feasible synthetic routes.
One-Step Synthesis Focus: Specifically designed for one-step synthesis, it provides concise and direct routes for your target compounds, streamlining the synthesis process.
Accurate Predictions: Utilizing the extensive PISTACHIO, BKMS_METABOLIC, PISTACHIO_RINGBREAKER, REAXYS, REAXYS_BIOCATALYSIS database, our tool offers high-accuracy predictions, reflecting the latest in chemical research and data.
Strategy Settings
Precursor scoring | Relevance Heuristic |
---|---|
Min. plausibility | 0.01 |
Model | Template_relevance |
Template Set | Pistachio/Bkms_metabolic/Pistachio_ringbreaker/Reaxys/Reaxys_biocatalysis |
Top-N result to add to graph | 6 |
Feasible Synthetic Routes
Q & A
Q1: What is the molecular formula, weight, and structure of triethyl orthoacetate?
A1: Triethyl orthoacetate has the molecular formula C8H18O3 and a molecular weight of 162.23 g/mol. Its structure consists of a central carbon atom bonded to three ethoxy groups (–OCH2CH3) and a methyl group (–CH3).
Q2: What is the Johnson-Claisen rearrangement, and how is triethyl orthoacetate involved?
A2: The Johnson-Claisen rearrangement is a [] useful reaction in organic chemistry that involves the thermal rearrangement of an allyl vinyl ether to produce a γ,δ-unsaturated carbonyl compound. Triethyl orthoacetate can react with allylic alcohols in the presence of an acid catalyst, forming an intermediate allyl vinyl ether, which then undergoes the Johnson-Claisen rearrangement []. This reaction offers a valuable method for synthesizing γ,δ-unsaturated esters, which are important building blocks for various organic molecules.
Q3: How can triethyl orthoacetate be used for the synthesis of heterocycles?
A3: Triethyl orthoacetate is a valuable reagent in the synthesis of various heterocyclic compounds [, , , , , , ]. For instance, it reacts with 2-aminonicotinamides in the presence of acetic acid to yield substituted pyrido[2,3-d]pyrimidines [].
Q4: Can triethyl orthoacetate be used for esterification reactions?
A4: Yes, triethyl orthoacetate can be employed as an alternative reagent for the esterification of carboxylic acids. This method, often carried out in ionic liquids, offers a milder and environmentally friendly approach compared to traditional methods using strong acids [].
Q5: How does the structure of triethyl orthoacetate influence its reactivity in the Johnson-Claisen rearrangement?
A5: The steric bulk of the ethoxy groups in triethyl orthoacetate can influence the stereochemical outcome of the Johnson-Claisen rearrangement []. The size of the orthoester employed (e.g., trimethyl orthoacetate vs. triethyl orthoacetate) can also impact the diastereoselectivity of the reaction [].
Q6: What analytical techniques are commonly used to characterize and quantify triethyl orthoacetate?
A7: Gas chromatography (GC) is a widely employed technique for the analysis of triethyl orthoacetate purity []. Other common techniques include nuclear magnetic resonance (NMR) spectroscopy and mass spectrometry (MS). These methods provide valuable information about the compound's structure, purity, and composition.
Q7: Are there any viable alternatives to triethyl orthoacetate in its various applications?
A9: Depending on the specific application, alternative reagents like other orthoesters (e.g., trimethyl orthoacetate, triethyl orthoformate) [], or different synthetic strategies might be considered.
Q8: What are potential future research directions for triethyl orthoacetate?
A8: Future research could focus on:
Disclaimer and Information on In-Vitro Research Products
Please be aware that all articles and product information presented on BenchChem are intended solely for informational purposes. The products available for purchase on BenchChem are specifically designed for in-vitro studies, which are conducted outside of living organisms. In-vitro studies, derived from the Latin term "in glass," involve experiments performed in controlled laboratory settings using cells or tissues. It is important to note that these products are not categorized as medicines or drugs, and they have not received approval from the FDA for the prevention, treatment, or cure of any medical condition, ailment, or disease. We must emphasize that any form of bodily introduction of these products into humans or animals is strictly prohibited by law. It is essential to adhere to these guidelines to ensure compliance with legal and ethical standards in research and experimentation.