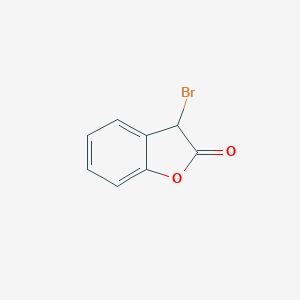
3-Bromo-2-coumaranone
Overview
Description
Nocistatin (bovine) is an endogenous peptide derived from the precursor protein prepronociceptin. It is known for its role in modulating pain perception by blocking the effects of nociceptin, another peptide derived from the same precursor. Nocistatin has been shown to inhibit nociceptin-induced allodynia and hyperalgesia, making it a significant compound in pain management research .
Mechanism of Action
Target of Action
It has been tested for allergenicity , suggesting that it may interact with immune cells or proteins involved in the immune response.
Mode of Action
It has been suggested that it may act as an allergen Allergens typically function by binding to immune cells or proteins, triggering an immune response
Biochemical Pathways
Given its potential role as an allergen , it may be involved in immune response pathways. These could include the activation of T cells, B cells, or other immune cells, leading to the production of antibodies and inflammation.
Pharmacokinetics
Its solubility in ethyl acetate suggests that it may be well-absorbed in the body, but further studies are needed to confirm this and to determine its distribution, metabolism, and excretion.
Result of Action
As a potential allergen , it may trigger an immune response leading to symptoms such as inflammation, redness, itching, and swelling
Action Environment
Environmental factors can significantly influence the action, efficacy, and stability of 3-Bromo-2-coumaranone. Factors such as temperature, pH, and the presence of other substances can affect its solubility, stability, and reactivity. For instance, it is recommended to be stored at a temperature of 2-8°C , suggesting that higher temperatures may affect its stability or efficacy
Preparation Methods
Synthetic Routes and Reaction Conditions: Nocistatin (bovine) can be synthesized using solid-phase peptide synthesis (SPPS), a common method for producing peptides. The process involves the sequential addition of protected amino acids to a growing peptide chain anchored to a solid resin. The reaction conditions typically include the use of coupling reagents like N,N’-diisopropylcarbodiimide (DIC) and hydroxybenzotriazole (HOBt) to facilitate peptide bond formation. The final peptide is cleaved from the resin using a mixture of trifluoroacetic acid (TFA) and scavengers to remove protecting groups .
Industrial Production Methods: Industrial production of nocistatin (bovine) involves large-scale SPPS, followed by purification using high-performance liquid chromatography (HPLC). The purified peptide is then lyophilized to obtain it in a stable, powdered form. The production process must adhere to stringent quality control measures to ensure the purity and consistency of the final product .
Chemical Reactions Analysis
Types of Reactions: Nocistatin (bovine) primarily undergoes hydrolysis and proteolysis. It can be cleaved by specific proteases to yield smaller peptide fragments, which may have distinct biological activities .
Common Reagents and Conditions:
Hydrolysis: Typically carried out under acidic or basic conditions.
Proteolysis: Enzymatic cleavage using proteases like trypsin or chymotrypsin.
Major Products: The major products formed from the hydrolysis and proteolysis of nocistatin (bovine) are smaller peptide fragments, which can modulate the activity of acid-sensing ion channels (ASICs) and other molecular targets .
Scientific Research Applications
Nocistatin (bovine) has a wide range of applications in scientific research:
Chemistry: Used as a model peptide for studying peptide synthesis and proteolysis.
Biology: Investigated for its role in modulating pain perception and its interactions with nociceptin.
Medicine: Explored as a potential therapeutic agent for pain management due to its ability to block nociceptin-induced hyperalgesia and allodynia.
Industry: Utilized in the development of new analgesic drugs and as a research tool in neuropharmacology
Comparison with Similar Compounds
Nociceptin: Another peptide derived from prepronociceptin, with opposing effects to nocistatin.
Dynorphin: An endogenous opioid peptide with analgesic properties.
Endorphins: Endogenous peptides that act on opioid receptors to modulate pain.
Comparison: Nocistatin (bovine) is unique in its ability to antagonize the effects of nociceptin, making it a valuable tool in pain research. Unlike nociceptin, which induces hyperalgesia and allodynia, nocistatin blocks these effects, highlighting its potential as a therapeutic agent for pain management .
Properties
IUPAC Name |
3-bromo-3H-1-benzofuran-2-one | |
---|---|---|
Source | PubChem | |
URL | https://pubchem.ncbi.nlm.nih.gov | |
Description | Data deposited in or computed by PubChem | |
InChI |
InChI=1S/C8H5BrO2/c9-7-5-3-1-2-4-6(5)11-8(7)10/h1-4,7H | |
Source | PubChem | |
URL | https://pubchem.ncbi.nlm.nih.gov | |
Description | Data deposited in or computed by PubChem | |
InChI Key |
MXSVEBOOHMKMQG-UHFFFAOYSA-N | |
Source | PubChem | |
URL | https://pubchem.ncbi.nlm.nih.gov | |
Description | Data deposited in or computed by PubChem | |
Canonical SMILES |
C1=CC=C2C(=C1)C(C(=O)O2)Br | |
Source | PubChem | |
URL | https://pubchem.ncbi.nlm.nih.gov | |
Description | Data deposited in or computed by PubChem | |
Molecular Formula |
C8H5BrO2 | |
Source | PubChem | |
URL | https://pubchem.ncbi.nlm.nih.gov | |
Description | Data deposited in or computed by PubChem | |
DSSTOX Substance ID |
DTXSID70393057 | |
Record name | 3-Bromo-2-coumaranone | |
Source | EPA DSSTox | |
URL | https://comptox.epa.gov/dashboard/DTXSID70393057 | |
Description | DSSTox provides a high quality public chemistry resource for supporting improved predictive toxicology. | |
Molecular Weight |
213.03 g/mol | |
Source | PubChem | |
URL | https://pubchem.ncbi.nlm.nih.gov | |
Description | Data deposited in or computed by PubChem | |
CAS No. |
115035-43-3 | |
Record name | 3-Bromo-2-coumaranone | |
Source | EPA DSSTox | |
URL | https://comptox.epa.gov/dashboard/DTXSID70393057 | |
Description | DSSTox provides a high quality public chemistry resource for supporting improved predictive toxicology. | |
Retrosynthesis Analysis
AI-Powered Synthesis Planning: Our tool employs the Template_relevance Pistachio, Template_relevance Bkms_metabolic, Template_relevance Pistachio_ringbreaker, Template_relevance Reaxys, Template_relevance Reaxys_biocatalysis model, leveraging a vast database of chemical reactions to predict feasible synthetic routes.
One-Step Synthesis Focus: Specifically designed for one-step synthesis, it provides concise and direct routes for your target compounds, streamlining the synthesis process.
Accurate Predictions: Utilizing the extensive PISTACHIO, BKMS_METABOLIC, PISTACHIO_RINGBREAKER, REAXYS, REAXYS_BIOCATALYSIS database, our tool offers high-accuracy predictions, reflecting the latest in chemical research and data.
Strategy Settings
Precursor scoring | Relevance Heuristic |
---|---|
Min. plausibility | 0.01 |
Model | Template_relevance |
Template Set | Pistachio/Bkms_metabolic/Pistachio_ringbreaker/Reaxys/Reaxys_biocatalysis |
Top-N result to add to graph | 6 |
Feasible Synthetic Routes
Q1: What is the significance of the Local Lymph Node Assay (LLNA) in the context of contact allergy research?
A1: The LLNA is a well-established method for assessing the skin sensitization potential of chemicals. [, ] Unlike traditional animal tests, the LLNA is an in vivo assay that measures the proliferation of lymphocytes in the lymph nodes draining the site of chemical application. This immune response is a key event in the development of allergic contact dermatitis (ACD).
Q2: How can SAR models be used in conjunction with the LLNA to improve the safety assessment of chemicals?
A2: Structure-activity relationship (SAR) models are computational tools that predict the toxicity of a chemical based on its molecular structure. [, ] By combining SAR models with the LLNA, researchers can screen a larger number of chemicals for their sensitization potential in a more efficient and cost-effective manner. This approach can help identify potential contact allergens early in the development process, allowing for the design of safer alternatives.
Disclaimer and Information on In-Vitro Research Products
Please be aware that all articles and product information presented on BenchChem are intended solely for informational purposes. The products available for purchase on BenchChem are specifically designed for in-vitro studies, which are conducted outside of living organisms. In-vitro studies, derived from the Latin term "in glass," involve experiments performed in controlled laboratory settings using cells or tissues. It is important to note that these products are not categorized as medicines or drugs, and they have not received approval from the FDA for the prevention, treatment, or cure of any medical condition, ailment, or disease. We must emphasize that any form of bodily introduction of these products into humans or animals is strictly prohibited by law. It is essential to adhere to these guidelines to ensure compliance with legal and ethical standards in research and experimentation.