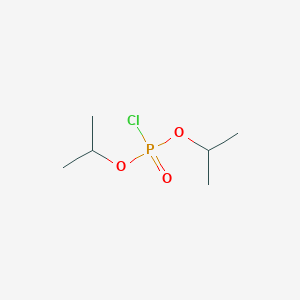
Diisopropyl chlorophosphate
Overview
Description
Diisopropyl chlorophosphate (DICP), with the chemical formula C₆H₁₄ClO₃P (CAS 2574-25-6), is an organophosphorus compound characterized by a central phosphorus atom bonded to two isopropyl groups, one chlorine atom, and an oxygen atom . DICP is widely used as a phosphorylating agent in organic synthesis, particularly in modifying nucleosides and synthesizing phosphate esters (e.g., in the preparation of 5’-phosphate diisopropyl esters of nucleosides with ~49% yield under pyridine conditions) .
DICP’s reactivity stems from its electrophilic phosphorus center, making it susceptible to nucleophilic substitution reactions. However, steric hindrance from the bulky isopropyl groups often reduces reaction rates compared to less hindered analogs .
Preparation Methods
Diisopropyl chlorophosphate can be synthesized through the reaction of diisopropyl phosphite with phosphorus trichloride under controlled conditions . The reaction typically involves the following steps:
Reaction of diisopropyl phosphite with phosphorus trichloride: This step generates diisopropyl phosphorochloridate.
Purification: The crude product is purified through distillation or recrystallization to obtain high-purity this compound.
Industrial production methods often involve similar synthetic routes but are optimized for large-scale production, ensuring high yield and purity.
Chemical Reactions Analysis
Diisopropyl chlorophosphate undergoes various chemical reactions, including:
Substitution Reactions: It can react with nucleophiles, such as amines or alcohols, to form corresponding phosphoramidates or phosphates.
Hydrolysis: In the presence of water, this compound hydrolyzes to form diisopropyl phosphate and hydrochloric acid.
Oxidation and Reduction: While not commonly involved in oxidation or reduction reactions, it can participate in such reactions under specific conditions.
Common reagents used in these reactions include amines, alcohols, and water. The major products formed depend on the specific reaction conditions and reagents used.
Scientific Research Applications
Organic Synthesis
DICP serves as a versatile reagent in organic synthesis, particularly in the preparation of phosphonates and phosphoramidates. Its ability to introduce phosphorus into organic molecules makes it valuable for creating compounds with potential biological activity. For instance, DICP can be used to synthesize phosphonylated derivatives that exhibit significant pharmacological properties.
Key Reactions Involving DICP:
- Phosphorylation Reactions: DICP is often employed to phosphorylate alcohols and amines, leading to the formation of phosphoric esters.
- Synthesis of Organophosphorus Compounds: It acts as a precursor for various organophosphorus compounds, which are important in agricultural and pharmaceutical industries.
Toxicological Studies
DICP is frequently used in toxicological research due to its structural similarity to nerve agents like sarin (GB). It acts as a simulant for studying the mechanisms of action of these potent neurotoxins, particularly their inhibition of acetylcholinesterase (AChE).
Applications in Toxicology:
- Inhibition Studies: DICP is utilized to evaluate the inhibitory effects on AChE, allowing researchers to understand the biochemical pathways affected by nerve agents.
- Decomposition Studies: Research has been conducted to analyze the decomposition pathways of DICP under various conditions, providing insights into the stability and reactivity of similar compounds in biological systems .
Kinetic Studies
The kinetics of DICP reactions have been extensively studied, particularly its anilinolysis and pyridinolysis mechanisms. These studies help elucidate the reaction pathways and rate constants associated with the hydrolysis and substitution reactions involving DICP.
Notable Kinetic Investigations:
- Anilinolysis in Acetonitrile: Research has demonstrated the kinetics and mechanisms involved when DICP reacts with aniline derivatives, providing valuable data on reaction rates and mechanisms .
- Pyridinolysis Mechanisms: Similar studies have been conducted focusing on pyridine derivatives reacting with DICP, highlighting differences in reaction rates based on nucleophile structure .
Coordination Chemistry
Recent studies have explored the interaction of DICP with coordination cage hosts. These investigations reveal that encapsulating DICP within coordination cages can significantly alter its hydrolysis rate, enhancing its stability in aqueous solutions. This application has implications for forensic sample preservation and analysis .
Research Findings:
- Stability Enhancement: The presence of coordination cages has been shown to protect DICP from hydrolysis, which is critical for maintaining sample integrity during forensic investigations.
- Host-Guest Chemistry: The binding interactions between DICP and various coordination cages have been characterized using NMR spectroscopy and crystallographic methods, revealing insights into its chemical behavior under different conditions .
Data Tables
Application Area | Specific Use Cases | Key Findings |
---|---|---|
Organic Synthesis | Phosphorylation of alcohols and amines | Effective reagent for introducing phosphorus groups |
Toxicological Studies | AChE inhibition studies | Simulant for studying nerve agent mechanisms |
Kinetic Studies | Anilinolysis and pyridinolysis | Detailed reaction mechanisms established |
Coordination Chemistry | Interaction with coordination cages | Enhanced stability against hydrolysis observed |
Case Studies
-
Toxicological Mechanism Analysis:
- Researchers utilized DICP to study AChE inhibition, revealing critical insights into the neurotoxic effects of organophosphates.
-
Kinetic Reaction Pathways:
- A detailed kinetic study on anilinolysis provided comprehensive data on reaction rates, contributing significantly to the understanding of organophosphate reactivity.
-
Forensic Sample Preservation:
- The encapsulation of DICP within coordination cages demonstrated a novel approach for preserving chemical samples in forensic settings, highlighting practical applications beyond traditional uses.
Mechanism of Action
The mechanism of action of diisopropyl chlorophosphate involves its ability to act as a phosphorylating agent. It can transfer its phosphoryl group to nucleophilic sites on target molecules, such as enzymes or other proteins. This phosphorylation can alter the activity of the target molecule, leading to various biochemical effects. The molecular targets and pathways involved depend on the specific application and context in which this compound is used.
Comparison with Similar Compounds
Comparison with Structurally Similar Compounds
Diisopropyl Fluorophosphate (DFP)
Structure : DFP (C₆H₁₄FO₃P) replaces DICP’s chlorine atom with fluorine.
Reactivity :
- DFP is more reactive toward cholinesterase enzymes due to fluorine’s superior leaving group ability. It irreversibly inhibits acetylcholinesterase (AChE) by phosphorylating the serine hydroxyl group, with 50% inhibition (IC₅₀) at 0.02 µM in vitro .
- In contrast, DICP exhibits weaker AChE inhibition (IC₅₀ ~10 µM) .
Toxicity : - DFP’s intraperitoneal LD₅₀ in mice is 0.05 mg/kg , significantly lower than DICP’s 2.5 mg/kg , highlighting DFP’s greater acute toxicity .
Applications : DFP is used in neuroscience research as a cholinesterase inhibitor, while DICP is primarily employed in synthetic chemistry .
Property | DICP | DFP |
---|---|---|
Leaving Group | Cl⁻ | F⁻ |
AChE IC₅₀ (µM) | ~10 | 0.02 |
Mouse LD₅₀ (mg/kg) | 2.5 | 0.05 |
Primary Use | Synthesis | Neurotoxin |
Diethyl Chlorophosphate (DCP)
Structure : DCP (C₄H₁₀ClO₃P) substitutes DICP’s isopropyl groups with ethyl groups.
Reactivity :
- DCP’s smaller ethyl groups reduce steric hindrance, enabling faster reaction kinetics. For example, DCP achieves 69% yield in nucleoside phosphorylation, outperforming DICP’s 49% yield under identical conditions .
Sensing Applications : - In Fe₂O₃ nanoparticle-based sensors, DCP induces a moderate reduction in magnetic moment, whereas DICP causes a significant reduction, suggesting stronger electron-withdrawing effects from the isopropyl groups . Toxicity: DCP’s LD₅₀ is higher than DICP’s, indicating lower acute toxicity .
Dimethyl Chlorophosphate (DMCP)
Structure : DMCP (C₂H₆ClO₃P) has methyl groups instead of isopropyl groups.
Reactivity :
- DMCP’s minimal steric hindrance allows rapid nucleophilic attacks. However, it is less stable than DICP due to weaker electron-donating effects from methyl groups.
Sensing : DMCP causes the largest reduction in Fe₂O₃ magnetic moment among chlorophosphates, attributed to its high electrophilicity .
Diisopropyl H-Phosphonate (DIPP)
Structure : DIPP (C₆H₁₅O₃P) replaces DICP’s chlorine with a hydroxyl group.
Reactivity :
- DIPP is less electrophilic due to the absence of a leaving group, making it more suitable for H-phosphonate coupling reactions rather than phosphorylation .
Applications : Used in oligonucleotide synthesis, contrasting with DICP’s role in phosphate ester formation .
Kinetic and Mechanistic Comparisons
- Anilinolysis in Acetonitrile: DICP reacts with aniline via a two-step mechanism (formation and breakdown of a pentacoordinate intermediate), with rate constants influenced by the nucleophile’s basicity .
- Serine Ester Phosphorylation: DICP phosphorylates serine methyl ester’s amino group in organic solvents, whereas DFP primarily targets hydroxyl groups .
Biological Activity
Diisopropyl chlorophosphate (DICP) is an organophosphorus compound that serves as a precursor in the synthesis of various organophosphate esters, including diisopropylfluorophosphate (DFP), which is known for its potent biological activity, particularly as an inhibitor of acetylcholinesterase (AChE). This article will explore the biological activity of DICP, focusing on its mechanism of action, toxicological effects, and relevant case studies.
The primary biological activity of DICP is linked to its role as a substrate for the synthesis of DFP, which inhibits AChE. AChE is an essential enzyme in the nervous system that catalyzes the breakdown of acetylcholine (ACh), a neurotransmitter. Inhibition of AChE leads to an accumulation of ACh at cholinergic synapses, resulting in prolonged stimulation of postsynaptic receptors, which can cause neurotoxicity and various physiological effects.
Key Points:
- AChE Inhibition : DICP acts as a precursor to DFP, which irreversibly binds to AChE.
- Neurotoxic Effects : The accumulation of ACh can lead to symptoms such as muscle twitching, respiratory failure, and potentially death if exposure is significant.
Toxicological Studies
Research has demonstrated the toxicological implications of DICP and its derivatives. For instance, studies involving zebrafish models have shown that exposure to DFP leads to significant mortality rates and reduced AChE activity in larvae. These findings underscore the compound's potential hazards when used in laboratory settings or encountered in environmental contexts.
Table 1: Toxicity Data from Zebrafish Studies
Concentration (mM) | Mortality Rate (%) | AChE Activity (% Control) |
---|---|---|
Control | 0 | 100 |
0.01 | 20 | 80 |
0.03 | 50 | 60 |
0.1 | 70 | 40 |
0.3 | 90 | 20 |
*Data adapted from multiple studies on DFP toxicity in zebrafish models .
Case Studies
-
Zebrafish Exposure Study :
In a controlled laboratory setting, zebrafish larvae were exposed to varying concentrations of DFP dissolved in dimethyl sulfoxide (DMSO). The study aimed to assess the mortality rates and changes in AChE activity over time. Results indicated that higher concentrations correlated with increased mortality and significantly decreased AChE activity compared to control groups . -
Environmental Impact Assessment :
Another study evaluated the environmental persistence and bioaccumulation potential of DICP and its derivatives. It was found that these compounds could volatilize and contaminate aquatic environments, posing risks to local wildlife and potentially entering human food chains through bioaccumulation . -
Synthesis and Stability Analysis :
Research on the synthesis and storage stability of DFP revealed that improper storage conditions could lead to degradation products that are less toxic than the parent compound. This finding emphasizes the importance of maintaining high purity levels for accurate toxicological evaluations .
Q & A
Basic Research Questions
Q. What are the recommended synthetic methodologies for preparing diisopropyl chlorophosphate in laboratory settings?
this compound can be synthesized via nucleophilic substitution reactions between phosphorus oxychloride (POCl₃) and isopropyl alcohol under controlled conditions. Key steps include maintaining anhydrous conditions and using a base (e.g., pyridine) to neutralize HCl byproducts. Reaction progress can be monitored via <sup>31</sup>P NMR spectroscopy to confirm the formation of the target compound and identify intermediates .
Q. Which spectroscopic techniques are most effective for characterizing this compound, and what spectral markers should researchers prioritize?
- <sup>31</sup>P NMR : Provides direct evidence of phosphorus-containing species; the chemical shift for this compound typically appears between δ +5 to +10 ppm.
- IR Spectroscopy : Look for P=O stretching vibrations (~1250–1300 cm⁻¹) and P–O–C linkages (~950–1050 cm⁻¹).
- Mass Spectrometry (ESI or EI) : Confirm molecular ion peaks (e.g., [M+H]⁺ or [M+Na]⁺) and fragmentation patterns specific to chlorophosphate esters .
Q. What safety protocols are essential for handling this compound in catalytic or kinetic studies?
- Personal Protective Equipment (PPE) : Use nitrile gloves, lab coats, and safety goggles. Avoid inhalation by working in a fume hood with adequate ventilation.
- Spill Management : Neutralize spills with inert adsorbents (e.g., vermiculite) and dispose of waste in sealed containers labeled for organophosphates.
- First Aid : Immediate flushing with water for skin/eye contact and medical consultation for exposure symptoms .
Advanced Research Questions
Q. How can molecular dynamics (MD) simulations predict the physicochemical behavior of this compound under varying temperatures and pressures?
MD simulations using force fields like GAFF or OPLS-AA can model density, compressibility, and diffusion coefficients. For example, studies on diisopropyl ether (a structural analog) revealed deviations of <5% between simulated and experimental densities at 303.15 K, highlighting the importance of validating potentials for chlorophosphate systems . Adjustments to partial charges or torsional parameters may improve accuracy for chlorinated derivatives.
Q. What mechanistic insights can kinetic studies provide for reactions involving this compound as a phosphorylating agent?
Pseudo-first-order kinetics under controlled pH and temperature conditions can elucidate nucleophilic substitution pathways. For instance, reactions with amines or alcohols may follow a two-step mechanism: (1) rapid formation of a trigonal bipyramidal intermediate and (2) rate-determining leaving-group expulsion. Isotopic labeling (<sup>18</sup>O in H₂O) or stopped-flow techniques can track intermediate lifetimes .
Q. How do metal-organic frameworks (MOFs) enhance the catalytic degradation of this compound, and what factors govern their efficiency?
MOFs like UiO-66 facilitate solvolysis via Lewis acid sites (e.g., Zr⁴⁺ nodes) that polarize P–Cl bonds. Key factors include:
- MOF Porosity : Higher surface area improves substrate access.
- Solvent Choice : Anhydrous methanol or ethanol accelerates degradation by stabilizing transition states.
- Temperature : Half-lives for simulants (e.g., diethyl chlorophosphate) decrease from 15 min at 25°C to 5.1 min at 40°C .
Q. What analytical challenges arise in resolving isomeric byproducts during this compound synthesis, and how can chromatography address them?
Isomeric impurities (e.g., mono- or tri-substituted phosphates) can co-elute in standard HPLC. Solutions include:
- Ion-Pair Chromatography : Use tetrabutylammonium salts to improve resolution of charged species.
- GC-MS with Derivatization : Trimethylsilyl derivatives enhance volatility for detecting trace isomers .
Q. Data Contradictions and Validation
- Computational vs. Experimental Density : MD simulations for diisopropyl ether overestimated experimental densities by ~3–5%, suggesting similar discrepancies may occur for chlorophosphates. Cross-validation with experimental data (e.g., pycnometry) is critical .
- Degradation Efficiency : MOF-based degradation rates for chlorophosphates vary widely (e.g., half-lives from 5 to 60 min) depending on solvent purity and MOF activation protocols. Reproducibility requires strict control of these parameters .
Properties
IUPAC Name |
2-[chloro(propan-2-yloxy)phosphoryl]oxypropane | |
---|---|---|
Source | PubChem | |
URL | https://pubchem.ncbi.nlm.nih.gov | |
Description | Data deposited in or computed by PubChem | |
InChI |
InChI=1S/C6H14ClO3P/c1-5(2)9-11(7,8)10-6(3)4/h5-6H,1-4H3 | |
Source | PubChem | |
URL | https://pubchem.ncbi.nlm.nih.gov | |
Description | Data deposited in or computed by PubChem | |
InChI Key |
GUFGWNUUDBGEGH-UHFFFAOYSA-N | |
Source | PubChem | |
URL | https://pubchem.ncbi.nlm.nih.gov | |
Description | Data deposited in or computed by PubChem | |
Canonical SMILES |
CC(C)OP(=O)(OC(C)C)Cl | |
Source | PubChem | |
URL | https://pubchem.ncbi.nlm.nih.gov | |
Description | Data deposited in or computed by PubChem | |
Molecular Formula |
C6H14ClO3P | |
Source | PubChem | |
URL | https://pubchem.ncbi.nlm.nih.gov | |
Description | Data deposited in or computed by PubChem | |
DSSTOX Substance ID |
DTXSID10180423 | |
Record name | Phosphorochloridic acid, bis(1-methylethyl) ester (9CI) | |
Source | EPA DSSTox | |
URL | https://comptox.epa.gov/dashboard/DTXSID10180423 | |
Description | DSSTox provides a high quality public chemistry resource for supporting improved predictive toxicology. | |
Molecular Weight |
200.60 g/mol | |
Source | PubChem | |
URL | https://pubchem.ncbi.nlm.nih.gov | |
Description | Data deposited in or computed by PubChem | |
CAS No. |
2574-25-6 | |
Record name | Bis(1-methylethyl) phosphorochloridate | |
Source | CAS Common Chemistry | |
URL | https://commonchemistry.cas.org/detail?cas_rn=2574-25-6 | |
Description | CAS Common Chemistry is an open community resource for accessing chemical information. Nearly 500,000 chemical substances from CAS REGISTRY cover areas of community interest, including common and frequently regulated chemicals, and those relevant to high school and undergraduate chemistry classes. This chemical information, curated by our expert scientists, is provided in alignment with our mission as a division of the American Chemical Society. | |
Explanation | The data from CAS Common Chemistry is provided under a CC-BY-NC 4.0 license, unless otherwise stated. | |
Record name | Phosphorochloridic acid, diisopropyl ester | |
Source | ChemIDplus | |
URL | https://pubchem.ncbi.nlm.nih.gov/substance/?source=chemidplus&sourceid=0002574256 | |
Description | ChemIDplus is a free, web search system that provides access to the structure and nomenclature authority files used for the identification of chemical substances cited in National Library of Medicine (NLM) databases, including the TOXNET system. | |
Record name | Phosphorochloridic acid, bis(1-methylethyl) ester (9CI) | |
Source | EPA DSSTox | |
URL | https://comptox.epa.gov/dashboard/DTXSID10180423 | |
Description | DSSTox provides a high quality public chemistry resource for supporting improved predictive toxicology. | |
Record name | Diisopropyl Phosphorochloridate | |
Source | European Chemicals Agency (ECHA) | |
URL | https://echa.europa.eu/information-on-chemicals | |
Description | The European Chemicals Agency (ECHA) is an agency of the European Union which is the driving force among regulatory authorities in implementing the EU's groundbreaking chemicals legislation for the benefit of human health and the environment as well as for innovation and competitiveness. | |
Explanation | Use of the information, documents and data from the ECHA website is subject to the terms and conditions of this Legal Notice, and subject to other binding limitations provided for under applicable law, the information, documents and data made available on the ECHA website may be reproduced, distributed and/or used, totally or in part, for non-commercial purposes provided that ECHA is acknowledged as the source: "Source: European Chemicals Agency, http://echa.europa.eu/". Such acknowledgement must be included in each copy of the material. ECHA permits and encourages organisations and individuals to create links to the ECHA website under the following cumulative conditions: Links can only be made to webpages that provide a link to the Legal Notice page. | |
Retrosynthesis Analysis
AI-Powered Synthesis Planning: Our tool employs the Template_relevance Pistachio, Template_relevance Bkms_metabolic, Template_relevance Pistachio_ringbreaker, Template_relevance Reaxys, Template_relevance Reaxys_biocatalysis model, leveraging a vast database of chemical reactions to predict feasible synthetic routes.
One-Step Synthesis Focus: Specifically designed for one-step synthesis, it provides concise and direct routes for your target compounds, streamlining the synthesis process.
Accurate Predictions: Utilizing the extensive PISTACHIO, BKMS_METABOLIC, PISTACHIO_RINGBREAKER, REAXYS, REAXYS_BIOCATALYSIS database, our tool offers high-accuracy predictions, reflecting the latest in chemical research and data.
Strategy Settings
Precursor scoring | Relevance Heuristic |
---|---|
Min. plausibility | 0.01 |
Model | Template_relevance |
Template Set | Pistachio/Bkms_metabolic/Pistachio_ringbreaker/Reaxys/Reaxys_biocatalysis |
Top-N result to add to graph | 6 |
Feasible Synthetic Routes
Disclaimer and Information on In-Vitro Research Products
Please be aware that all articles and product information presented on BenchChem are intended solely for informational purposes. The products available for purchase on BenchChem are specifically designed for in-vitro studies, which are conducted outside of living organisms. In-vitro studies, derived from the Latin term "in glass," involve experiments performed in controlled laboratory settings using cells or tissues. It is important to note that these products are not categorized as medicines or drugs, and they have not received approval from the FDA for the prevention, treatment, or cure of any medical condition, ailment, or disease. We must emphasize that any form of bodily introduction of these products into humans or animals is strictly prohibited by law. It is essential to adhere to these guidelines to ensure compliance with legal and ethical standards in research and experimentation.