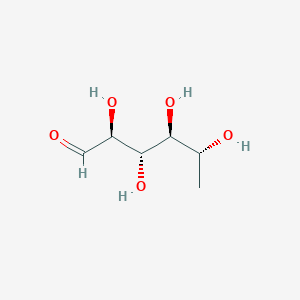
6-Deoxy-L-talose
Overview
Description
6-Deoxy-L-talose is an aldohexose sugar that is found in the cell wall of several microorganisms . It was first isolated from polysaccharides in the cell wall of Actinomyces bovis . Furthermore, this aldohexose sugar is a C-2 epimer of galactose .
Synthesis Analysis
The synthesis of 6-Deoxy-L-talose has been achieved through various methods. A [3 + 2] block glycosylation strategy was used for the synthesis of a pentasaccharide repeating unit of Acinetobacter baumannii K11 capsular polysaccharides . Another approach involved the isomerization of 6-deoxy D-tagatose, which was produced from D-fucose .
Molecular Structure Analysis
The molecular formula of 6-Deoxy-L-talose is C6H12O5 . It has a molecular weight of 164.16 g/mol . The active sugar form of this compound is dTDP-6-deoxy-L-talose .
Chemical Reactions Analysis
All 6-deoxyhexose and aldohexose isomers favored the 4C1 chair conformation, while oxidation at C-6 showed a shift in equilibrium to favor the 1C4 chair for β-alluronic acid, β-guluronic acid, and β-iduronic acid . The anomeric preference was found to be significantly affected by a remote change in oxidation state, with the alternate anomer favored for several isomers .
Physical And Chemical Properties Analysis
6-Deoxy-L-talose is a solid substance . It has a molecular weight of 164.16 g/mol . Its solubility varies with different solvents: DMF: 10mg/mL, DMSO: 20mg/mL, PBS (pH 7.2): 10mg/mL .
Scientific Research Applications
Heparan Sulfate Mimetics
6-Deoxy-L-talose has been investigated as a potential building block for heparan sulfate (HS) mimetics. HS is a complex glycosaminoglycan involved in various biological processes, including cell signaling, angiogenesis, and inflammation. Researchers have synthesized disaccharides containing 6-deoxy-α-L-talose to mimic specific HS disaccharide motifs. These mimetics aim to inhibit angiogenesis by blocking the formation of the ternary complex between HS, fibroblast growth factors (FGFs), and their receptors, which is crucial for tumor growth and development .
Natural Product Glycosides
Streptomyces sp. strain GoM1 produces novel glycosides containing 6-deoxy-α-L-talose. These compounds exhibit diverse aglycones, such as small phenols, isovaleric acid, or aromatic carboxylic acids. The biosynthesis of these glycosides can be enhanced through precursor-directed approaches .
Acinetobacter baumannii Capsular Polysaccharides
A concise total synthesis of a pentasaccharide repeating unit from Acinetobacter baumannii K11 capsular polysaccharides involves 6-deoxy-L-talose. This rare sugar is part of the pentasaccharide structure, and its synthesis employs a [3 + 2] block glycosylation strategy .
Enzymatic Epimerization
Enzymes capable of epimerizing l-hexoses, including 6-deoxy-L-talose, have been studied. For instance, these enzymes can catalyze the conversion between l-sorbose and l-tagatose or between l-psicose and l-fructose .
Mechanism of Action
Target of Action
6-Deoxy-L-Talose is a microbial monosaccharide that has been found in the cell wall of bacteria such as S. bovis and in the O-specific polysaccharide of LPS in species including E. coli and P. maltophilia . It is also a component of the surface glycopeptidolipids of M. avium . Therefore, its primary targets are these bacterial cell walls and surface glycopeptidolipids.
Mode of Action
These interactions lead to changes in the structure and function of the bacterial cell walls and surface glycopeptidolipids .
Biochemical Pathways
The biosynthesis of 6-Deoxy-L-Talose involves several biochemical pathways. The chemical reduction of GDP-4-keto-6-deoxy-D-mannose leads to the formation of GDP-D-rhamnose and GDP-6-deoxy-D-talose . This process involves various enzymes and intermediates, and it affects downstream effects related to the structure and function of bacterial cell walls and surface glycopeptidolipids .
Pharmacokinetics
It is known that it is a solid compound with a molecular weight of 1642 and it is soluble in DMF, DMSO, and PBS (pH 72) .
Result of Action
The result of the action of 6-Deoxy-L-Talose is the alteration of the structure and function of bacterial cell walls and surface glycopeptidolipids . This can potentially affect the virulence and survival of the bacteria.
Future Directions
The synthesis of 6-Deoxy-L-talose is a challenging task and has been achieved using different approaches . The target oligosaccharide is attractive for further glycoconjugate formation with a suitable aglycon . This provides a platform to test steric and electronic effects of pyranose substituents, with the goal of formulating straightforward rules that govern carbohydrate reactivity and drive quicker, more efficient syntheses .
properties
IUPAC Name |
(2R,3R,4R,5S)-2,3,4,5-tetrahydroxyhexanal | |
---|---|---|
Source | PubChem | |
URL | https://pubchem.ncbi.nlm.nih.gov | |
Description | Data deposited in or computed by PubChem | |
InChI |
InChI=1S/C6H12O5/c1-3(8)5(10)6(11)4(9)2-7/h2-6,8-11H,1H3/t3-,4-,5+,6-/m0/s1 | |
Source | PubChem | |
URL | https://pubchem.ncbi.nlm.nih.gov | |
Description | Data deposited in or computed by PubChem | |
InChI Key |
PNNNRSAQSRJVSB-AZGQCCRYSA-N | |
Source | PubChem | |
URL | https://pubchem.ncbi.nlm.nih.gov | |
Description | Data deposited in or computed by PubChem | |
Canonical SMILES |
CC(C(C(C(C=O)O)O)O)O | |
Source | PubChem | |
URL | https://pubchem.ncbi.nlm.nih.gov | |
Description | Data deposited in or computed by PubChem | |
Isomeric SMILES |
C[C@@H]([C@H]([C@H]([C@H](C=O)O)O)O)O | |
Source | PubChem | |
URL | https://pubchem.ncbi.nlm.nih.gov | |
Description | Data deposited in or computed by PubChem | |
Molecular Formula |
C6H12O5 | |
Source | PubChem | |
URL | https://pubchem.ncbi.nlm.nih.gov | |
Description | Data deposited in or computed by PubChem | |
Molecular Weight |
164.16 g/mol | |
Source | PubChem | |
URL | https://pubchem.ncbi.nlm.nih.gov | |
Description | Data deposited in or computed by PubChem | |
Product Name |
6-Deoxy-L-talose |
Synthesis routes and methods
Procedure details
Retrosynthesis Analysis
AI-Powered Synthesis Planning: Our tool employs the Template_relevance Pistachio, Template_relevance Bkms_metabolic, Template_relevance Pistachio_ringbreaker, Template_relevance Reaxys, Template_relevance Reaxys_biocatalysis model, leveraging a vast database of chemical reactions to predict feasible synthetic routes.
One-Step Synthesis Focus: Specifically designed for one-step synthesis, it provides concise and direct routes for your target compounds, streamlining the synthesis process.
Accurate Predictions: Utilizing the extensive PISTACHIO, BKMS_METABOLIC, PISTACHIO_RINGBREAKER, REAXYS, REAXYS_BIOCATALYSIS database, our tool offers high-accuracy predictions, reflecting the latest in chemical research and data.
Strategy Settings
Precursor scoring | Relevance Heuristic |
---|---|
Min. plausibility | 0.01 |
Model | Template_relevance |
Template Set | Pistachio/Bkms_metabolic/Pistachio_ringbreaker/Reaxys/Reaxys_biocatalysis |
Top-N result to add to graph | 6 |
Feasible Synthetic Routes
Q & A
Q1: What is the molecular formula and weight of 6-Deoxy-L-talose?
A1: 6-Deoxy-L-talose has the molecular formula C6H12O5 and a molecular weight of 164.16 g/mol.
Q2: What spectroscopic data is available for 6-Deoxy-L-talose?
A2: Researchers frequently utilize Nuclear Magnetic Resonance (NMR) spectroscopy, including 1H NMR and 13C NMR, to characterize the structure of 6-Deoxy-L-talose-containing compounds. [, , , , , , ] Mass spectrometry techniques like FAB-MS, ESI-MS, and MALDI-TOF are also employed for structural analysis. [, , , ]
Q3: How is 6-Deoxy-L-talose synthesized in bacteria?
A3: The biosynthesis of 6-Deoxy-L-talose often starts with deoxy-thymidine diphosphate (dTDP)-D-glucose, which undergoes a series of enzymatic transformations, including epimerization and reduction, to yield dTDP-6-Deoxy-L-talose. [, ]
Q4: Which bacteria are known to produce 6-Deoxy-L-talose?
A4: Several bacterial species, including Actinomyces viscosus, Actinobacillus actinomycetemcomitans, Burkholderia pseudomallei, Pseudomonas fluorescens, Yokenella regensburgei, and Streptococcus bovis, have been found to incorporate 6-Deoxy-L-talose into their cell wall structures or produce it as part of exopolysaccharides. [, , , , , , , , , , , ]
Q5: How does 6-Deoxy-L-talose contribute to the antigenicity of bacterial lipopolysaccharides (LPS)?
A5: 6-Deoxy-L-talose is often a component of the O-antigen, a highly variable region of LPS responsible for serotype specificity. Variations in the linkage and substitution patterns of 6-Deoxy-L-talose within the O-antigen contribute to the antigenic diversity observed in bacteria. [, , , , , , , , ]
Q6: Does the acetylation of 6-Deoxy-L-talose influence its biological activity?
A6: Yes, O-acetylation of 6-Deoxy-L-talose can significantly impact the biological activity of LPS. For instance, in Burkholderia pseudomallei, specific O-acetyl modifications on 6-Deoxy-L-talose residues are recognized by monoclonal antibodies and influence the colony morphology of the bacteria. [, ]
Q7: Are there any known interactions between 6-Deoxy-L-talose and mammalian cells?
A7: Research suggests that a protein-associated agglutinin found in plant seed-derived D-mannose might interact with 6-Deoxy-L-talose in the cell walls of Actinomyces viscosus and Actinomyces naeslundii. [] This interaction could potentially play a role in bacterial adhesion to mammalian cells.
Q8: Can 6-Deoxy-L-talose be chemically synthesized?
A8: Yes, several synthetic routes to 6-Deoxy-L-talose have been developed, often starting from readily available sugars like L-rhamnose. [, , , , , , , ]
Q9: What are the potential applications of synthetic 6-Deoxy-L-talose?
A9: Synthetic 6-Deoxy-L-talose and its derivatives are valuable tools for studying bacterial polysaccharide biosynthesis, exploring structure-activity relationships in LPS, and developing vaccines and diagnostic tools for bacterial infections. [, ]
Q10: What are some key areas for future research on 6-Deoxy-L-talose?
A10: Future research could focus on:
- Detailed characterization of enzymes involved in 6-Deoxy-L-talose biosynthesis: This would facilitate the development of novel antibacterial agents targeting these pathways. [, , ]
- Elucidating the precise role of 6-Deoxy-L-talose in bacterial physiology: This could include investigating its involvement in cell wall integrity, biofilm formation, and host-pathogen interactions. []
- Developing synthetic methodologies for producing 6-Deoxy-L-talose-containing oligosaccharides: This would enable the creation of well-defined structures for studying their immunological properties and developing glycoconjugate vaccines. [, ]
- Investigating the potential of 6-Deoxy-L-talose-based molecules as therapeutic agents: For example, they could be explored for their anti-cancer or immunomodulatory activities. []
Disclaimer and Information on In-Vitro Research Products
Please be aware that all articles and product information presented on BenchChem are intended solely for informational purposes. The products available for purchase on BenchChem are specifically designed for in-vitro studies, which are conducted outside of living organisms. In-vitro studies, derived from the Latin term "in glass," involve experiments performed in controlled laboratory settings using cells or tissues. It is important to note that these products are not categorized as medicines or drugs, and they have not received approval from the FDA for the prevention, treatment, or cure of any medical condition, ailment, or disease. We must emphasize that any form of bodily introduction of these products into humans or animals is strictly prohibited by law. It is essential to adhere to these guidelines to ensure compliance with legal and ethical standards in research and experimentation.