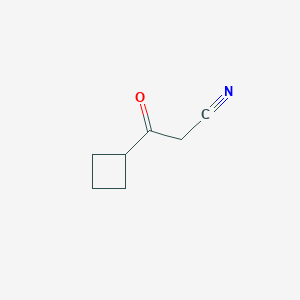
3-Cyclobutyl-3-oxopropanenitrile
Overview
Description
3-Cyclobutyl-3-oxopropanenitrile is an organic compound with the molecular formula C7H9NO. It is known for its unique structure, which includes a cyclobutyl ring attached to a nitrile group and a ketone group. This compound is used in various chemical reactions and has applications in scientific research and industry .
Preparation Methods
Synthetic Routes and Reaction Conditions
The preparation of 3-Cyclobutyl-3-oxopropanenitrile typically involves the reaction of cyclobutane propionitrile with alcohol under acid catalysis to generate esterification products. These products are then hydrolyzed under alkali catalysis to obtain this compound .
Industrial Production Methods
In industrial settings, the synthesis of this compound may involve bulk manufacturing processes that ensure high purity and yield. The compound is often produced in sealed, dry conditions and stored at low temperatures to maintain its stability .
Chemical Reactions Analysis
Types of Reactions
3-Cyclobutyl-3-oxopropanenitrile undergoes various types of chemical reactions, including:
Esterification: Reaction with alcohols under acid catalysis.
Acylation: Introduction of an acyl group into the molecule.
Amination: Addition of an amine group.
Addition Reactions: Reactions where atoms or groups are added to the molecule.
Common Reagents and Conditions
Common reagents used in these reactions include acids for catalysis, alkalis for hydrolysis, and various organic solvents. The conditions often involve controlled temperatures and pressures to ensure the desired reaction outcomes .
Major Products Formed
The major products formed from these reactions depend on the specific reagents and conditions used. For example, esterification can produce esters, while acylation can introduce acyl groups into the molecule .
Scientific Research Applications
3-Cyclobutyl-3-oxopropanenitrile has a wide range of applications in scientific research, including:
Chemistry: Used as an intermediate in organic synthesis and as a solvent.
Biology: Investigated for its potential biological activities and interactions with biomolecules.
Medicine: Explored for its potential therapeutic properties and as a building block for drug development.
Industry: Utilized in the production of various chemicals and materials
Mechanism of Action
The mechanism of action of 3-Cyclobutyl-3-oxopropanenitrile involves its interaction with specific molecular targets and pathways. The compound can participate in various chemical reactions, influencing the behavior of other molecules and potentially leading to desired biological or chemical effects .
Comparison with Similar Compounds
Similar Compounds
Cyclobutanepropanenitrile: Similar structure but lacks the ketone group.
Cyclobutyl-oxo-propionitrile: Another related compound with slight structural differences.
Uniqueness
3-Cyclobutyl-3-oxopropanenitrile is unique due to its combination of a cyclobutyl ring, nitrile group, and ketone group. This unique structure allows it to participate in a variety of chemical reactions and makes it valuable in scientific research and industrial applications .
Properties
IUPAC Name |
3-cyclobutyl-3-oxopropanenitrile | |
---|---|---|
Source | PubChem | |
URL | https://pubchem.ncbi.nlm.nih.gov | |
Description | Data deposited in or computed by PubChem | |
InChI |
InChI=1S/C7H9NO/c8-5-4-7(9)6-2-1-3-6/h6H,1-4H2 | |
Source | PubChem | |
URL | https://pubchem.ncbi.nlm.nih.gov | |
Description | Data deposited in or computed by PubChem | |
InChI Key |
BAJYSJUMDLMTGH-UHFFFAOYSA-N | |
Source | PubChem | |
URL | https://pubchem.ncbi.nlm.nih.gov | |
Description | Data deposited in or computed by PubChem | |
Canonical SMILES |
C1CC(C1)C(=O)CC#N | |
Source | PubChem | |
URL | https://pubchem.ncbi.nlm.nih.gov | |
Description | Data deposited in or computed by PubChem | |
Molecular Formula |
C7H9NO | |
Source | PubChem | |
URL | https://pubchem.ncbi.nlm.nih.gov | |
Description | Data deposited in or computed by PubChem | |
DSSTOX Substance ID |
DTXSID50551370 | |
Record name | 3-Cyclobutyl-3-oxopropanenitrile | |
Source | EPA DSSTox | |
URL | https://comptox.epa.gov/dashboard/DTXSID50551370 | |
Description | DSSTox provides a high quality public chemistry resource for supporting improved predictive toxicology. | |
Molecular Weight |
123.15 g/mol | |
Source | PubChem | |
URL | https://pubchem.ncbi.nlm.nih.gov | |
Description | Data deposited in or computed by PubChem | |
CAS No. |
118431-89-3 | |
Record name | 3-Cyclobutyl-3-oxopropanenitrile | |
Source | EPA DSSTox | |
URL | https://comptox.epa.gov/dashboard/DTXSID50551370 | |
Description | DSSTox provides a high quality public chemistry resource for supporting improved predictive toxicology. | |
Synthesis routes and methods I
Procedure details
Synthesis routes and methods II
Procedure details
Synthesis routes and methods III
Procedure details
Synthesis routes and methods IV
Procedure details
Disclaimer and Information on In-Vitro Research Products
Please be aware that all articles and product information presented on BenchChem are intended solely for informational purposes. The products available for purchase on BenchChem are specifically designed for in-vitro studies, which are conducted outside of living organisms. In-vitro studies, derived from the Latin term "in glass," involve experiments performed in controlled laboratory settings using cells or tissues. It is important to note that these products are not categorized as medicines or drugs, and they have not received approval from the FDA for the prevention, treatment, or cure of any medical condition, ailment, or disease. We must emphasize that any form of bodily introduction of these products into humans or animals is strictly prohibited by law. It is essential to adhere to these guidelines to ensure compliance with legal and ethical standards in research and experimentation.