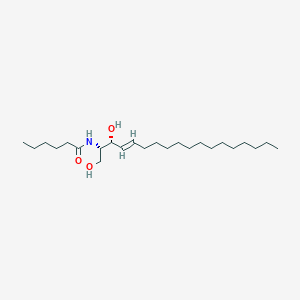
N-hexanoylsphingosine
Overview
Description
N-hexanoylsphingosine, also known as Ceramide C6, is a chemical compound with the molecular formula C24H47NO3 . It is currently being evaluated as an antineoplastic agent, after preclinical studies showing its property to reduce tumor growth .
Synthesis Analysis
Cells actively metabolize exogenously administered N-hexanoylsphingosine (C6-Cer) to natural (i.e., long-chain) ceramide (LC-Cer) via the sphingosine (Sph) salvage pathway, namely via C6-Cer deacylation and Sph reacylation with a long-chain fatty acid .Molecular Structure Analysis
The molecular structure of N-hexanoylsphingosine consists of 24 carbon atoms, 47 hydrogen atoms, 1 nitrogen atom, and 3 oxygen atoms . The average mass is 397.635 Da, and the monoisotopic mass is 397.355591 Da .Physical And Chemical Properties Analysis
N-hexanoylsphingosine has a density of 0.9±0.1 g/cm3, a boiling point of 574.7±50.0 °C at 760 mmHg, and a flash point of 301.4±30.1 °C . It has 4 H bond acceptors, 3 H bond donors, and 20 freely rotating bonds .Scientific Research Applications
Cancer Therapy: Canine Mammary Cancer
C6 ceramide has shown promise as a tumor suppressor in the treatment of canine mammary cancer. It inhibits cell growth, migration, and invasion in vitro and decreases tumor growth and metastasis in vivo. The compound targets EGR3 , a promoter of cancer cell proliferation and migration, by modulating the JAK1/STAT3 signaling pathway .
Cutaneous T Cell Lymphoma Treatment
In the context of cutaneous T cell lymphoma (CTCL), C6 ceramide has been evaluated as a novel treatment option. It significantly reduces cell viability and induces cell death via apoptosis and necrosis in CTCL cell lines, while having minimal effects on healthy keratinocytes .
Neurotumor Cells: Apoptosis and Metabolic Downregulation
C6 ceramide’s cytotoxic effects on neurotumor cells have been linked to its ability to maintain elevated intracellular levels, despite rapid metabolic removal. It achieves this by impinging on the sphingosine salvage pathway , leading to the accumulation of natural ceramide, which lacks apoptotic properties in these cells .
Sphingolipid Anticancer Therapeutics
As an antineoplastic agent, C6 ceramide is being explored for its potential to reduce tumor growth. Its metabolism is driven by direct glucosylation and utilization via the sphingosine salvage pathway, which is relevant for optimizing its therapeutic use .
Drug Delivery Systems: Complexation with Cholesteryl Phosphocholine
C6 ceramide complexed with cholesteryl phosphocholine (CholPC) has been developed as a potent, solvent-free delivery formulation for cells in culture. This complexation increases the bioavailability of ceramide for cells and potentiates its effects compared to solvent-dissolved C6 ceramide .
Proangiogenic Activity Inhibition in Multiple Myeloma
Research has indicated that C6 ceramide treatment can inhibit the proangiogenic activity of exosomes in multiple myeloma. This suggests a potential application in preventing the formation of new blood vessels that support tumor growth and metastasis .
Mechanism of Action
Target of Action
C6 Ceramide, also known as N-hexanoylsphingosine or C6-ceramide, primarily targets the ceramide pathway . The ceramide pathway plays a crucial role in regulating cellular metabolism . Ceramides are synthesized by a family of six ceramide synthase enzymes (CerS), each with distinct specificity for the acyl-CoA substrate . The CerS family is expressed in a variety of human tumors and is involved in tumorigenesis .
Mode of Action
C6 Ceramide interacts with its targets and induces changes in cellular metabolism. It has been found that C6 Ceramide competes with C24-ceramide for the binding site of phosphatidylinositol-5-phosphate-4-kinase type-2 gamma (PIP4K2C) . This interaction leads to the deactivation of multiple oncogenic signaling pathways and promotes cell death .
Biochemical Pathways
C6 Ceramide affects several biochemical pathways. Ceramides and dihydroceramides are synthesized by CerS enzymes via a ping-pong reaction mechanism involving a covalent acyl-enzyme intermediate . Ceramides can also be used as the attachment site of phosphorylation or phosphocholine to generate ceramide-1-phosphate or sphingomyelin . The hydrolysis of sphingomyelin can also produce ceramides .
Pharmacokinetics
The pharmacokinetics of C6 Ceramide involves its absorption, distribution, metabolism, and excretion (ADME). The maximum concentration and area under the curve increase with dose . Clearance is consistent between doses and is observed mainly through the liver without significant hepatotoxicity . The half-life ranges from 20 to 30 hours and the volume of distribution is consistent with a lipophilic drug .
Result of Action
C6 Ceramide shows activity against a variety of cancer cell lines . It can inhibit cell proliferation and induce apoptosis . It also permeates cell membranes and causes mitochondrial Ca2+ influx more efficiently than C6 Ceramide in DMSO .
Action Environment
The action, efficacy, and stability of C6 Ceramide can be influenced by environmental factors. For instance, the formulation used to deliver C6 Ceramide to cells can significantly impact its bioavailability and potency . C6 Ceramide complexed with Cholesteryl Phosphocholine led to much larger biological effects in cultured cells compared to C6 Ceramide dissolved in dimethyl sulfoxide (DMSO) .
properties
IUPAC Name |
N-[(E,2S,3R)-1,3-dihydroxyoctadec-4-en-2-yl]hexanamide | |
---|---|---|
Source | PubChem | |
URL | https://pubchem.ncbi.nlm.nih.gov | |
Description | Data deposited in or computed by PubChem | |
InChI |
InChI=1S/C24H47NO3/c1-3-5-7-8-9-10-11-12-13-14-15-16-18-19-23(27)22(21-26)25-24(28)20-17-6-4-2/h18-19,22-23,26-27H,3-17,20-21H2,1-2H3,(H,25,28)/b19-18+/t22-,23+/m0/s1 | |
Source | PubChem | |
URL | https://pubchem.ncbi.nlm.nih.gov | |
Description | Data deposited in or computed by PubChem | |
InChI Key |
NPRJSFWNFTXXQC-QFWQFVLDSA-N | |
Source | PubChem | |
URL | https://pubchem.ncbi.nlm.nih.gov | |
Description | Data deposited in or computed by PubChem | |
Canonical SMILES |
CCCCCCCCCCCCCC=CC(C(CO)NC(=O)CCCCC)O | |
Source | PubChem | |
URL | https://pubchem.ncbi.nlm.nih.gov | |
Description | Data deposited in or computed by PubChem | |
Isomeric SMILES |
CCCCCCCCCCCCC/C=C/[C@H]([C@H](CO)NC(=O)CCCCC)O | |
Source | PubChem | |
URL | https://pubchem.ncbi.nlm.nih.gov | |
Description | Data deposited in or computed by PubChem | |
Molecular Formula |
C24H47NO3 | |
Source | PubChem | |
URL | https://pubchem.ncbi.nlm.nih.gov | |
Description | Data deposited in or computed by PubChem | |
DSSTOX Substance ID |
DTXSID90924923 | |
Record name | N-Hexanoylsphingosine | |
Source | EPA DSSTox | |
URL | https://comptox.epa.gov/dashboard/DTXSID90924923 | |
Description | DSSTox provides a high quality public chemistry resource for supporting improved predictive toxicology. | |
Molecular Weight |
397.6 g/mol | |
Source | PubChem | |
URL | https://pubchem.ncbi.nlm.nih.gov | |
Description | Data deposited in or computed by PubChem | |
Product Name |
N-hexanoylsphingosine | |
CAS RN |
124753-97-5 | |
Record name | N-Hexanoylsphingosine | |
Source | ChemIDplus | |
URL | https://pubchem.ncbi.nlm.nih.gov/substance/?source=chemidplus&sourceid=0124753975 | |
Description | ChemIDplus is a free, web search system that provides access to the structure and nomenclature authority files used for the identification of chemical substances cited in National Library of Medicine (NLM) databases, including the TOXNET system. | |
Record name | N-Hexanoylsphingosine | |
Source | EPA DSSTox | |
URL | https://comptox.epa.gov/dashboard/DTXSID90924923 | |
Description | DSSTox provides a high quality public chemistry resource for supporting improved predictive toxicology. | |
Record name | N-HEXANOYLSPHINGOSINE | |
Source | FDA Global Substance Registration System (GSRS) | |
URL | https://gsrs.ncats.nih.gov/ginas/app/beta/substances/038753E78J | |
Description | The FDA Global Substance Registration System (GSRS) enables the efficient and accurate exchange of information on what substances are in regulated products. Instead of relying on names, which vary across regulatory domains, countries, and regions, the GSRS knowledge base makes it possible for substances to be defined by standardized, scientific descriptions. | |
Explanation | Unless otherwise noted, the contents of the FDA website (www.fda.gov), both text and graphics, are not copyrighted. They are in the public domain and may be republished, reprinted and otherwise used freely by anyone without the need to obtain permission from FDA. Credit to the U.S. Food and Drug Administration as the source is appreciated but not required. | |
Retrosynthesis Analysis
AI-Powered Synthesis Planning: Our tool employs the Template_relevance Pistachio, Template_relevance Bkms_metabolic, Template_relevance Pistachio_ringbreaker, Template_relevance Reaxys, Template_relevance Reaxys_biocatalysis model, leveraging a vast database of chemical reactions to predict feasible synthetic routes.
One-Step Synthesis Focus: Specifically designed for one-step synthesis, it provides concise and direct routes for your target compounds, streamlining the synthesis process.
Accurate Predictions: Utilizing the extensive PISTACHIO, BKMS_METABOLIC, PISTACHIO_RINGBREAKER, REAXYS, REAXYS_BIOCATALYSIS database, our tool offers high-accuracy predictions, reflecting the latest in chemical research and data.
Strategy Settings
Precursor scoring | Relevance Heuristic |
---|---|
Min. plausibility | 0.01 |
Model | Template_relevance |
Template Set | Pistachio/Bkms_metabolic/Pistachio_ringbreaker/Reaxys/Reaxys_biocatalysis |
Top-N result to add to graph | 6 |
Feasible Synthetic Routes
Disclaimer and Information on In-Vitro Research Products
Please be aware that all articles and product information presented on BenchChem are intended solely for informational purposes. The products available for purchase on BenchChem are specifically designed for in-vitro studies, which are conducted outside of living organisms. In-vitro studies, derived from the Latin term "in glass," involve experiments performed in controlled laboratory settings using cells or tissues. It is important to note that these products are not categorized as medicines or drugs, and they have not received approval from the FDA for the prevention, treatment, or cure of any medical condition, ailment, or disease. We must emphasize that any form of bodily introduction of these products into humans or animals is strictly prohibited by law. It is essential to adhere to these guidelines to ensure compliance with legal and ethical standards in research and experimentation.