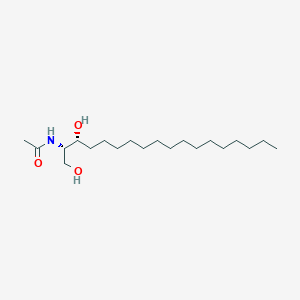
C2 Dihydroceramide
Overview
Description
C2 Dihydroceramide is a sphingolipid characterized by a saturated acyl chain (lacking the 4–5 double bond found in ceramide) and an amide-linked fatty acid at carbon 2 of the sphingoid base . It is a precursor in the de novo sphingolipid biosynthesis pathway and has traditionally been used as a negative control in apoptosis studies due to its perceived biological inertness . However, recent research demonstrates that this compound exhibits unique bioactivities, including stimulation of ABCA1-mediated cholesterol efflux, modulation of lipid signaling in neuropathic pain, and selective cytotoxicity in cancer cells .
Preparation Methods
Chemical Synthesis Approaches
Starting Materials and Reaction Design
The chemical synthesis of C2 dihydroceramide begins with D-erythro-sphinganine (2-amino-1,3-dihydroxyoctadecane) as the sphingoid backbone. The C2 fatty acid, typically an acetyl group, is introduced via N-acylation. Key reagents include acetyl chloride or acetic anhydride for acylation, and protecting groups such as tert-butyldimethylsilyl (TBDMS) or benzyl ethers to shield hydroxyl groups during reaction conditions . The synthesis proceeds through three stages:
-
Protection : Hydroxyl groups at C1 and C3 are protected to prevent undesired esterification.
-
Acylation : The primary amine at C2 reacts with acetyl chloride in the presence of a base (e.g., triethylamine) to form the amide bond.
-
Deprotection : Acidic or catalytic hydrogenation conditions remove protecting groups, yielding free hydroxyls .
Stereochemical Considerations
Natural this compound exists exclusively in the D-erythro stereoisomer form. Synthetic routes must enforce this configuration to avoid non-biological isoforms (D-threo, L-erythro, L-threo). Chiral auxiliaries or enantioselective catalysis during sphinganine synthesis ensure stereochemical fidelity. For example, Sharpless epoxidation or enzymatic resolution can achieve >98% enantiomeric excess .
Purification and Characterization
Post-synthesis purification employs silica gel chromatography with gradients of chloroform/methanol/water (65:25:4, v/v) to isolate the product. Thin-layer chromatography (TLC) using a solvent system of chloroform/methanol/ammonia (80:20:2, v/v) confirms purity (98% by TLC) . Nuclear magnetic resonance (NMR) spectroscopy validates structure:
-
¹H NMR : Peaks at δ 0.88 (t, 3H, terminal CH₃), δ 1.25 (m, 26H, alkyl chain), δ 2.02 (s, 3H, acetyl CH₃), δ 3.85 (m, 1H, C3-OH).
Enzymatic and Biological Production Methods
In Vitro Enzymatic Synthesis
Ceramide synthase (CerS) isoforms catalyze the acyl-CoA-dependent N-acylation of sphinganine. For this compound, recombinant CerS2 (specific for short-chain acyl-CoAs) is incubated with sphinganine and acetyl-CoA in a buffer containing 50 mM HEPES (pH 7.4), 5 mM MgCl₂, and 0.1% Triton X-100. Reactions proceed at 37°C for 2–4 hours, achieving ~60% conversion efficiency .
Cellular Biosynthesis with Desaturase Inhibitors
Inhibiting dihydroceramide desaturase (DES1) blocks the conversion of dihydroceramide to ceramide, enabling intracellular accumulation. Cells (e.g., SMS-KCNR neuroblastoma) are treated with cyclopropanated inhibitors (e.g., 10 μM GT-11) and fed sphinganine (20 μM) and sodium acetate (5 mM). Lipids are extracted using chloroform/methanol (2:1, v/v) and purified via reversed-phase HPLC (C18 column, acetonitrile/water gradient) .
Comparative Analysis of Synthesis Methodologies
Parameter | Chemical Synthesis | Enzymatic Synthesis | Biological Accumulation |
---|---|---|---|
Yield | 70–85% | 55–65% | 10–20% (cell-based) |
Purity | ≥98% (TLC) | 90–95% (HPLC) | 80–90% (HPLC) |
Stereoselectivity | High (chiral control) | Moderate (enzyme-specific) | Low (requires purification) |
Scalability | Industrial-scale feasible | Lab-scale only | Limited by cell culture |
Cost | High (reagents, catalysts) | Moderate (enzyme costs) | Low (endogenous pathways) |
Analytical Techniques for Quality Assurance
Mass Spectrometry
High-resolution mass spectrometry (HRMS) confirms molecular weight (343.56 g/mol) with an observed [M+H]⁺ ion at m/z 344.2698 (calculated 344.2701) . Fragmentation patterns show characteristic losses of H₂O (−18 Da) and the acetyl group (−60 Da).
Solubility and Stability
This compound exhibits solubility in ethanol (5 mg/mL) and DMSO (5 mg/mL). Stability studies indicate a 12-month shelf life at −20°C in anhydrous conditions, with degradation <2% .
Chemical Reactions Analysis
Types of Reactions
N-acetylsphinganine undergoes various chemical reactions, including:
Oxidation: It can be oxidized to form N-acetylsphingosine.
Reduction: Reduction reactions can convert it back to sphinganine.
Substitution: The acetyl group can be substituted with other acyl groups to form different derivatives.
Common Reagents and Conditions
Oxidation: Common oxidizing agents include hydrogen peroxide and potassium permanganate.
Reduction: Reducing agents such as lithium aluminum hydride are used.
Substitution: Acyl chlorides and anhydrides are commonly used for substitution reactions.
Major Products Formed
Oxidation: N-acetylsphingosine.
Reduction: Sphinganine.
Substitution: Various N-acylsphinganine derivatives.
Scientific Research Applications
Metabolic Studies
Recent studies have begun to elucidate the role of dihydroceramides in metabolic pathways, particularly concerning insulin sensitivity and diabetes:
- Insulin Resistance : Research indicates that C2 ceramide can induce insulin resistance through the salvage/recycling pathway in muscle cells. Although C2 dihydroceramide itself does not directly affect insulin signaling, its relationship with ceramides suggests that it may play a role in metabolic dysregulation when converted to active forms .
- Cardiometabolic Disease Risk : Dihydroceramides have been linked to cardiometabolic diseases such as type 2 diabetes (T2D) and cardiovascular disease (CVD). Profiling studies have shown that specific dihydroceramides correlate with increased risk factors for these conditions. For instance, certain dihydroceramides (e.g., dhCer20:0) are associated with higher T2D risk, while others (e.g., dhCer22:2) have been linked to increased CVD risk .
Cancer Research
This compound's role in cancer biology has also been investigated:
- Apoptosis Induction : While C2 ceramide has been shown to induce apoptosis in various cancer cell lines, studies indicate that this compound lacks this effect. This distinction is crucial for understanding the specific mechanisms through which ceramides influence cell death pathways .
- Cell Growth Inhibition : The selectivity of ceramide-mediated biology highlights that while C2 ceramide effectively inhibits cell growth and induces apoptosis, this compound does not exhibit these properties, making it a useful control for experimental designs .
Immunological Applications
Research has indicated that short-chain ceramides, including this compound, can disrupt immunoreceptor signaling:
- Immunoreceptor Function : Studies demonstrate that short-chain ceramides can affect phase separation within cellular membranes, potentially influencing immune responses. This disruption could have implications for understanding immune-related diseases and therapies .
Data Tables and Case Studies
The following tables summarize key findings related to the applications of this compound across different research areas:
Mechanism of Action
N-acetylsphinganine exerts its effects by interacting with cellular membranes and influencing sphingolipid metabolism. It can modulate the activity of enzymes involved in sphingolipid biosynthesis and degradation. The compound also affects signaling pathways related to cell growth and apoptosis, making it a potential therapeutic agent for various diseases .
Comparison with Similar Compounds
Structural Analog: C2 Ceramide
C2 Ceramide differs from C2 Dihydroceramide by the presence of a 4–5 double bond in the sphingoid base. Despite this structural variance, both compounds share similar cellular uptake kinetics .
Key Insight : The saturated acyl chain in this compound eliminates apoptotic side effects while retaining cholesterol efflux capabilities, making it a safer candidate for cardiovascular therapies .
Chain-Length Variants: C6, C8, and C16 Dihydroceramides
Dihydroceramides with longer acyl chains exhibit distinct biological roles:
Mechanistic Divergence : Chain length influences subcellular localization and target specificity. For example, C16 Dihydroceramide accumulates in nervous tissue, modulating TRPV1 channels, whereas shorter-chain analogs primarily affect lipid metabolism .
Branched-Chain Dihydroceramide Analogs
Branched alkyl chains enhance cytotoxicity and selectivity in cancer cells. For example, compound 4 (15DES-N-acyl11) exhibits:
- 5-fold higher cytotoxicity in SKBR-3 breast cancer cells compared to C2 Ceramide .
- Improved selectivity index (SI = 3) over non-cancerous cells (e.g., NHDF fibroblasts) .
Structural Advantage : Branching increases lipophilicity and membrane penetration, enhancing pro-apoptotic signaling .
Functional Antagonists: Ceramide and Sphingosine-1-Phosphate (S1P)
Pathway Crosstalk : Dihydroceramide’s effects are context-dependent, balancing ceramide-mediated apoptosis and S1P-driven survival .
Therapeutic Implications
Biological Activity
C2 dihydroceramide, a sphingolipid derivative, has garnered attention in recent years for its potential biological activities and implications in various health conditions. Unlike its counterpart, C2 ceramide, which is well-documented for its role in apoptosis and cellular signaling, this compound has been historically regarded as biologically inactive. This article provides a comprehensive overview of the biological activity of this compound, supported by recent research findings, data tables, and case studies.
Overview of Dihydroceramides
Dihydroceramides are structural analogs of ceramides that lack the double bond between the 4th and 5th carbon atoms of the sphingoid backbone. This structural difference significantly impacts their biological functions. Early studies suggested that dihydroceramides were inert; however, more recent research indicates that they may play roles in metabolic processes and disease states.
1. Apoptosis and Cell Growth
Research has demonstrated that this compound does not induce apoptosis in transformed human keratinocytes or colon cancer cells, contrasting sharply with C2 ceramide, which is known to promote cell death through various mechanisms. For instance, studies show that while C2 ceramide effectively triggers apoptosis and cell cycle arrest at the G2/M phase, this compound fails to elicit similar responses in human cancer cell lines such as HCT-116 .
Table 1: Comparison of Biological Effects of C2 Ceramide vs. This compound
Biological Activity | C2 Ceramide | This compound |
---|---|---|
Induces Apoptosis | Yes | No |
Cell Cycle Arrest (G2/M phase) | Yes | No |
Effects on Cell Viability | Decreases | No significant effect |
2. Metabolic Implications
Recent studies have linked dihydroceramides with metabolic disorders such as Type 2 Diabetes (T2D) and cardiovascular diseases (CVD). Specifically, certain dihydroceramides have been associated with increased risk factors for these conditions. For example, dihydroceramides C20:0 and C22:2 are correlated with higher T2D risk, while others like C26:1 show protective effects against T2D .
Table 2: Association of Dihydroceramides with Disease Risk
Dihydroceramide | Associated Risk |
---|---|
dhCer20:0 | Higher T2D risk |
dhCer22:0 | Higher T2D risk |
dhCer26:1 | Lower T2D risk |
dhCer22:2 | Higher CVD risk |
The mechanisms underlying the biological inactivity of this compound compared to ceramides are not fully understood. It is hypothesized that the absence of the double bond affects its ability to activate certain signaling pathways involved in apoptosis and cell growth regulation. For instance, this compound does not activate ceramide-activated protein phosphatase, which may explain its lack of apoptotic activity .
Case Study 1: Insulin Sensitivity
A study investigating the effects of ceramides on insulin sensitivity found that while C2 ceramide induced a loss in insulin sensitivity through alterations in lipid metabolism in muscle cells, this compound did not exhibit similar effects. This highlights the distinct roles these compounds play in metabolic regulation .
Case Study 2: Cardiovascular Health
In a nested case-cohort study involving participants at risk for cardiovascular disease, specific dihydroceramides were linked to increased cardiovascular risk factors. This suggests that while this compound may not be directly active in apoptosis or cell growth regulation, it could contribute to broader metabolic dysregulation impacting heart health .
Q & A
Basic Research Questions
Q. What is the structural and functional role of C2 Dihydroceramide in sphingolipid metabolism?
this compound (d18:0/2:0) is a short-chain sphingolipid precursor that lacks the 4,5-double bond present in ceramides. It serves as a substrate for dihydroceramide desaturases (DES1/DES2), which catalyze its conversion to bioactive ceramides . Methodologically, its role in cholesterol efflux can be studied using assays measuring ABCA1 transporter activity, as this compound stimulates ABCA1-mediated cholesterol removal from cells . Researchers often employ radiolabeled cholesterol or fluorescent lipid analogs to quantify efflux efficiency.
Q. How is this compound synthesized and characterized in experimental models?
Synthetic this compound is typically produced via acylation of sphinganine (d18:0) with a short-chain fatty acid (e.g., acetic acid for the C2 variant). Structural validation requires nuclear magnetic resonance (NMR) spectroscopy and mass spectrometry (MS) to confirm acyl chain length and saturation status . For in vitro studies, it is often dissolved in organic solvents (e.g., ethanol or DMSO) and delivered to cells via lipid carriers like bovine serum albumin (BSA) to ensure solubility .
Q. Why is this compound frequently used as a negative control in ceramide studies?
Unlike ceramides, this compound lacks the 4,5-trans double bond, rendering it incapable of forming the membrane microdomains critical for apoptotic signaling. However, researchers must validate its inertness in specific contexts, as some studies report weak pro-apoptotic effects at high concentrations (e.g., in P388 cells), necessitating dose-response assays and comparison with fully saturated analogs .
Advanced Research Questions
Q. How do dihydroceramide desaturases (DES1/DES2) regulate the ceramide/dihydroceramide ratio, and what are the metabolic implications?
DES1 introduces the 4,5-double bond to convert dihydroceramide to ceramide, a process localized to the endoplasmic reticulum . Inhibition of DES1 (e.g., via siRNA or small molecules like GT11) elevates dihydroceramide levels, which in adipose tissue models reduces ceramide/dihydroceramide ratios and improves insulin sensitivity . Advanced studies combine lipidomics (LC-MS/MS) with isotopic tracing to monitor flux through the pathway, particularly in obesity or non-alcoholic fatty liver disease (NAFLD) models .
Q. What experimental strategies resolve contradictions in this compound bioactivity across studies?
Discrepancies arise from cell type-specific responses, concentration thresholds, and off-target effects. For example, in Jurkat cells, dihydroceramide accumulation via siRNA-mediated suppression of sphingomyelin synthase 1 (SMS1) enhances photodynamic therapy (PDT) sensitivity, measured via LC-MS-based quantification of C22-/C24-dihydroceramides . Researchers must standardize delivery methods (e.g., lipid carriers vs. direct solvent exposure) and include multiple controls (e.g., scrambled siRNA, DES1 knockout models) to isolate dihydroceramide-specific effects .
Q. How does this compound influence mitochondrial function and oxidative stress?
While ceramides directly impair mitochondrial electron transport, this compound exhibits weaker effects. However, in neuronal models, DES1 inhibition increases dihydroceramide levels, which sequesters Rac1 in the cytosol, exacerbating oxidative stress. This can be assessed via Seahorse assays (mitochondrial respiration) and ROS-sensitive dyes (e.g., DCFH-DA) . Co-treatment with antioxidants (e.g., NAC) helps distinguish dihydroceramide-specific ROS contributions .
Q. What methodologies are optimal for quantifying this compound in complex biological samples?
High-resolution mass spectrometry (HR-MS) with multiple reaction monitoring (MRM) is the gold standard. For tissue samples, lipid extraction via Folch or Bligh-Dyer methods is followed by normalization to internal standards (e.g., C17-dihydroceramide). Data should be reported as molar ratios to total protein or phospholipid content to account for matrix variability .
Q. How do tissue-specific ceramide/dihydroceramide ratios impact translational research?
In human NAFLD cohorts, hepatic dihydroceramide accumulation correlates with mitochondrial dysfunction but not insulin resistance, contrasting findings in murine models. Cross-tissue lipidomic profiling (liver, muscle, adipose) via LC-MS/MS reveals organ-specific sphingolipid metabolism, necessitating stratified analyses in preclinical-to-clinical translation .
Q. Methodological Considerations
- Experimental Design : Include dose-response curves and genetic/pharmacological DES1 modulation to dissect dihydroceramide-specific effects .
- Data Interpretation : Use multivariate statistics (e.g., PCA) to address lipidomic dataset complexity and avoid overinterpreting correlative findings .
- Controls : Pair this compound with fully inert analogs (e.g., C2 phytoceramide) to distinguish chain length vs. saturation effects .
Properties
IUPAC Name |
N-[(2S,3R)-1,3-dihydroxyoctadecan-2-yl]acetamide | |
---|---|---|
Source | PubChem | |
URL | https://pubchem.ncbi.nlm.nih.gov | |
Description | Data deposited in or computed by PubChem | |
InChI |
InChI=1S/C20H41NO3/c1-3-4-5-6-7-8-9-10-11-12-13-14-15-16-20(24)19(17-22)21-18(2)23/h19-20,22,24H,3-17H2,1-2H3,(H,21,23)/t19-,20+/m0/s1 | |
Source | PubChem | |
URL | https://pubchem.ncbi.nlm.nih.gov | |
Description | Data deposited in or computed by PubChem | |
InChI Key |
CRJGESKKUOMBCT-VQTJNVASSA-N | |
Source | PubChem | |
URL | https://pubchem.ncbi.nlm.nih.gov | |
Description | Data deposited in or computed by PubChem | |
Canonical SMILES |
CCCCCCCCCCCCCCCC(C(CO)NC(=O)C)O | |
Source | PubChem | |
URL | https://pubchem.ncbi.nlm.nih.gov | |
Description | Data deposited in or computed by PubChem | |
Isomeric SMILES |
CCCCCCCCCCCCCCC[C@H]([C@H](CO)NC(=O)C)O | |
Source | PubChem | |
URL | https://pubchem.ncbi.nlm.nih.gov | |
Description | Data deposited in or computed by PubChem | |
Molecular Formula |
C20H41NO3 | |
Source | PubChem | |
URL | https://pubchem.ncbi.nlm.nih.gov | |
Description | Data deposited in or computed by PubChem | |
DSSTOX Substance ID |
DTXSID20156452 | |
Record name | N-Acetyl dihydrosphingosine | |
Source | EPA DSSTox | |
URL | https://comptox.epa.gov/dashboard/DTXSID20156452 | |
Description | DSSTox provides a high quality public chemistry resource for supporting improved predictive toxicology. | |
Molecular Weight |
343.5 g/mol | |
Source | PubChem | |
URL | https://pubchem.ncbi.nlm.nih.gov | |
Description | Data deposited in or computed by PubChem | |
CAS No. |
13031-64-6 | |
Record name | N-[(1S,2R)-2-Hydroxy-1-(hydroxymethyl)heptadecyl]acetamide | |
Source | CAS Common Chemistry | |
URL | https://commonchemistry.cas.org/detail?cas_rn=13031-64-6 | |
Description | CAS Common Chemistry is an open community resource for accessing chemical information. Nearly 500,000 chemical substances from CAS REGISTRY cover areas of community interest, including common and frequently regulated chemicals, and those relevant to high school and undergraduate chemistry classes. This chemical information, curated by our expert scientists, is provided in alignment with our mission as a division of the American Chemical Society. | |
Explanation | The data from CAS Common Chemistry is provided under a CC-BY-NC 4.0 license, unless otherwise stated. | |
Record name | N-Acetyl dihydrosphingosine | |
Source | ChemIDplus | |
URL | https://pubchem.ncbi.nlm.nih.gov/substance/?source=chemidplus&sourceid=0013031646 | |
Description | ChemIDplus is a free, web search system that provides access to the structure and nomenclature authority files used for the identification of chemical substances cited in National Library of Medicine (NLM) databases, including the TOXNET system. | |
Record name | N-Acetyl dihydrosphingosine | |
Source | EPA DSSTox | |
URL | https://comptox.epa.gov/dashboard/DTXSID20156452 | |
Description | DSSTox provides a high quality public chemistry resource for supporting improved predictive toxicology. | |
Record name | N-ACETYL DIHYDROSPHINGOSINE | |
Source | FDA Global Substance Registration System (GSRS) | |
URL | https://gsrs.ncats.nih.gov/ginas/app/beta/substances/ZZK8X1CR0R | |
Description | The FDA Global Substance Registration System (GSRS) enables the efficient and accurate exchange of information on what substances are in regulated products. Instead of relying on names, which vary across regulatory domains, countries, and regions, the GSRS knowledge base makes it possible for substances to be defined by standardized, scientific descriptions. | |
Explanation | Unless otherwise noted, the contents of the FDA website (www.fda.gov), both text and graphics, are not copyrighted. They are in the public domain and may be republished, reprinted and otherwise used freely by anyone without the need to obtain permission from FDA. Credit to the U.S. Food and Drug Administration as the source is appreciated but not required. | |
Synthesis routes and methods
Procedure details
Retrosynthesis Analysis
AI-Powered Synthesis Planning: Our tool employs the Template_relevance Pistachio, Template_relevance Bkms_metabolic, Template_relevance Pistachio_ringbreaker, Template_relevance Reaxys, Template_relevance Reaxys_biocatalysis model, leveraging a vast database of chemical reactions to predict feasible synthetic routes.
One-Step Synthesis Focus: Specifically designed for one-step synthesis, it provides concise and direct routes for your target compounds, streamlining the synthesis process.
Accurate Predictions: Utilizing the extensive PISTACHIO, BKMS_METABOLIC, PISTACHIO_RINGBREAKER, REAXYS, REAXYS_BIOCATALYSIS database, our tool offers high-accuracy predictions, reflecting the latest in chemical research and data.
Strategy Settings
Precursor scoring | Relevance Heuristic |
---|---|
Min. plausibility | 0.01 |
Model | Template_relevance |
Template Set | Pistachio/Bkms_metabolic/Pistachio_ringbreaker/Reaxys/Reaxys_biocatalysis |
Top-N result to add to graph | 6 |
Feasible Synthetic Routes
Disclaimer and Information on In-Vitro Research Products
Please be aware that all articles and product information presented on BenchChem are intended solely for informational purposes. The products available for purchase on BenchChem are specifically designed for in-vitro studies, which are conducted outside of living organisms. In-vitro studies, derived from the Latin term "in glass," involve experiments performed in controlled laboratory settings using cells or tissues. It is important to note that these products are not categorized as medicines or drugs, and they have not received approval from the FDA for the prevention, treatment, or cure of any medical condition, ailment, or disease. We must emphasize that any form of bodily introduction of these products into humans or animals is strictly prohibited by law. It is essential to adhere to these guidelines to ensure compliance with legal and ethical standards in research and experimentation.