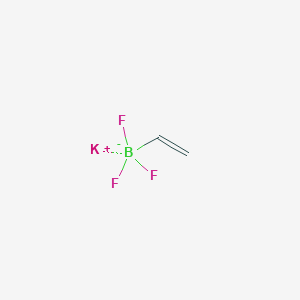
Potassium vinyltrifluoroborate
Overview
Description
LX-2931 is a small molecule inhibitor of sphingosine-1-phosphate lyase, an enzyme involved in the metabolism of sphingosine-1-phosphate. This compound has been investigated for its potential therapeutic applications in treating autoimmune diseases, particularly rheumatoid arthritis .
Mechanism of Action
Target of Action
Potassium vinyltrifluoroborate is a versatile organometallic reagent . Its primary targets are organic compounds that undergo coupling reactions . It is used as a vinylating agent in the presence of palladium catalysts .
Mode of Action
The compound interacts with its targets through a process known as the Suzuki-Miyaura cross-coupling reaction . This reaction involves the coupling of an aryl or vinyl boronic acid with an aryl or vinyl halide catalyzed by a palladium(0) complex .
Biochemical Pathways
This compound affects several biochemical pathways. It is involved in Suzuki-Miyaura cross-coupling reactions and polymerization reactions . It also plays a role in the synthesis of photonic crystals and sensitizers for dye-sensitized solar cells . Furthermore, it is used in Mannich/diastereoselective hydroamination reaction sequences .
Pharmacokinetics
It is known to be air- and water-stable , which suggests that it may have good stability in various environments.
Result of Action
The result of this compound’s action is the formation of new organic compounds through coupling reactions . These reactions can lead to the creation of complex organic molecules, which are often used in the development of pharmaceuticals and other chemical products .
Action Environment
The action of this compound can be influenced by various environmental factors. For instance, the presence of a palladium catalyst is necessary for the compound to act as a vinylating agent . Additionally, the compound is stable in both air and water , suggesting that it can maintain its efficacy in a variety of environmental conditions.
Biochemical Analysis
Biochemical Properties
The biochemical properties of Potassium Vinyltrifluoroborate are largely tied to its role as a vinylating agent. It interacts with various biomolecules in the presence of palladium catalysts . The nature of these interactions is largely dependent on the specific biomolecules and reaction conditions involved .
Cellular Effects
Given its role in biochemical reactions, it is likely that it influences cell function through its interactions with various biomolecules .
Molecular Mechanism
The molecular mechanism of this compound involves its role as a vinylating agent. It exerts its effects at the molecular level through binding interactions with biomolecules in the presence of palladium catalysts .
Temporal Effects in Laboratory Settings
It is known to be stable and can be utilized in coupling reactions under relatively mild conditions .
Preparation Methods
The synthetic route for LX-2931 involves multiple steps, starting from commercially available starting materials. The key steps include the formation of an imidazole ring and the introduction of hydroxyl groups. The reaction conditions typically involve the use of organic solvents and catalysts to facilitate the formation of the desired product. Industrial production methods may involve optimization of these synthetic routes to improve yield and purity .
Chemical Reactions Analysis
LX-2931 undergoes various chemical reactions, including:
Oxidation: The compound can be oxidized under specific conditions to form corresponding oxides.
Reduction: Reduction reactions can be carried out to modify the functional groups present in the compound.
Substitution: LX-2931 can undergo substitution reactions where one functional group is replaced by another. Common reagents used in these reactions include oxidizing agents like hydrogen peroxide, reducing agents like sodium borohydride, and various catalysts.
Scientific Research Applications
Chemistry: Used as a tool compound to study sphingosine-1-phosphate metabolism.
Biology: Investigated for its effects on cellular signaling pathways involving sphingosine-1-phosphate.
Medicine: Explored as a therapeutic agent for autoimmune diseases, particularly rheumatoid arthritis.
Industry: Potential applications in the development of new drugs targeting sphingosine-1-phosphate lyase.
Comparison with Similar Compounds
LX-2931 is unique in its specific inhibition of sphingosine-1-phosphate lyase. Similar compounds include:
Fingolimod: A sphingosine-1-phosphate receptor modulator used in the treatment of multiple sclerosis.
Siponimod: Another sphingosine-1-phosphate receptor modulator with applications in multiple sclerosis.
Properties
IUPAC Name |
potassium;ethenyl(trifluoro)boranuide | |
---|---|---|
Source | PubChem | |
URL | https://pubchem.ncbi.nlm.nih.gov | |
Description | Data deposited in or computed by PubChem | |
InChI |
InChI=1S/C2H3BF3.K/c1-2-3(4,5)6;/h2H,1H2;/q-1;+1 | |
Source | PubChem | |
URL | https://pubchem.ncbi.nlm.nih.gov | |
Description | Data deposited in or computed by PubChem | |
InChI Key |
ZCUMGICZWDOJEM-UHFFFAOYSA-N | |
Source | PubChem | |
URL | https://pubchem.ncbi.nlm.nih.gov | |
Description | Data deposited in or computed by PubChem | |
Canonical SMILES |
[B-](C=C)(F)(F)F.[K+] | |
Source | PubChem | |
URL | https://pubchem.ncbi.nlm.nih.gov | |
Description | Data deposited in or computed by PubChem | |
Molecular Formula |
C2H3BF3K | |
Source | PubChem | |
URL | https://pubchem.ncbi.nlm.nih.gov | |
Description | Data deposited in or computed by PubChem | |
DSSTOX Substance ID |
DTXSID40635557 | |
Record name | Potassium ethenyl(trifluoro)borate(1-) | |
Source | EPA DSSTox | |
URL | https://comptox.epa.gov/dashboard/DTXSID40635557 | |
Description | DSSTox provides a high quality public chemistry resource for supporting improved predictive toxicology. | |
Molecular Weight |
133.95 g/mol | |
Source | PubChem | |
URL | https://pubchem.ncbi.nlm.nih.gov | |
Description | Data deposited in or computed by PubChem | |
CAS No. |
13682-77-4 | |
Record name | Potassium ethenyl(trifluoro)borate(1-) | |
Source | EPA DSSTox | |
URL | https://comptox.epa.gov/dashboard/DTXSID40635557 | |
Description | DSSTox provides a high quality public chemistry resource for supporting improved predictive toxicology. | |
Record name | Potassium vinyltrifluoroborate | |
Source | European Chemicals Agency (ECHA) | |
URL | https://echa.europa.eu/information-on-chemicals | |
Description | The European Chemicals Agency (ECHA) is an agency of the European Union which is the driving force among regulatory authorities in implementing the EU's groundbreaking chemicals legislation for the benefit of human health and the environment as well as for innovation and competitiveness. | |
Explanation | Use of the information, documents and data from the ECHA website is subject to the terms and conditions of this Legal Notice, and subject to other binding limitations provided for under applicable law, the information, documents and data made available on the ECHA website may be reproduced, distributed and/or used, totally or in part, for non-commercial purposes provided that ECHA is acknowledged as the source: "Source: European Chemicals Agency, http://echa.europa.eu/". Such acknowledgement must be included in each copy of the material. ECHA permits and encourages organisations and individuals to create links to the ECHA website under the following cumulative conditions: Links can only be made to webpages that provide a link to the Legal Notice page. | |
Disclaimer and Information on In-Vitro Research Products
Please be aware that all articles and product information presented on BenchChem are intended solely for informational purposes. The products available for purchase on BenchChem are specifically designed for in-vitro studies, which are conducted outside of living organisms. In-vitro studies, derived from the Latin term "in glass," involve experiments performed in controlled laboratory settings using cells or tissues. It is important to note that these products are not categorized as medicines or drugs, and they have not received approval from the FDA for the prevention, treatment, or cure of any medical condition, ailment, or disease. We must emphasize that any form of bodily introduction of these products into humans or animals is strictly prohibited by law. It is essential to adhere to these guidelines to ensure compliance with legal and ethical standards in research and experimentation.