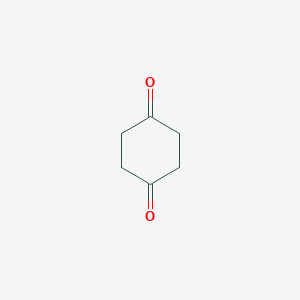
1,4-Cyclohexanedione
Overview
Description
1,4-Cyclohexanedione (C₆H₈O₂; IUPAC name: cyclohexane-1,4-dione) is a six-membered cyclic diketone with ketone groups at the 1 and 4 positions. Its molecular weight is 112.13 g/mol, and it exhibits a planar chair conformation stabilized by conjugation between the carbonyl groups . Key properties include:
- Melting Point: 76–80°C
- Solubility: Soluble in ethanol, chloroform, and water; insoluble in diethyl ether and petroleum ether .
- Synthesis: Common methods include (1) oxidation of 1,4-cyclohexanediol using hydrogen peroxide with sodium tungstate , and (2) catalytic dehydrogenation of cyclohexanediols over Pd/C in structured reactors (yield: 47.7% at 300°C) .
This compound is a critical intermediate in pharmaceuticals (e.g., frovatriptan for migraines ), organic synthesis (e.g., precursors for TCNQ, a strong electron acceptor ), and heterocyclic chemistry (e.g., fused dibenzophenanthrolines ).
Preparation Methods
Catalytic Hydrogenation Followed by Oxidation
Hydrogenation of Hydroquinone to 1,4-Cyclohexanediol
The most industrially viable route begins with hydroquinone, which undergoes catalytic hydrogenation to produce 1,4-cyclohexanediol. A skeleton nickel catalyst, aqueous sodium hydroxide, and water serve as the reaction medium under hydrogen pressures of 1.0–1.2 MPa at 150°C . This step achieves yields of 85–90% with minimal byproducts, attributed to the catalyst’s stability and recyclability .
Reaction Conditions:
-
Catalyst: Skeleton nickel (6 g per 56 g hydroquinone)
-
Solvent: Water (250 mL)
-
Temperature: 150°C
-
Pressure: 1.0–1.2 MPa H₂
Oxidation of 1,4-Cyclohexanediol to 1,4-Cyclohexanedione
The diol intermediate is oxidized to the dione using hydrogen peroxide (H₂O₂) under catalytic conditions. Sodium tungstate or quaternary ammonium decatungstate, paired with ligands like oxalic acid, enables efficient oxidation at 40–120°C over 12–48 hours . This method avoids toxic solvents, leveraging water as the primary medium, and achieves yields of 78–79% .
Optimized Protocol:
-
Catalyst: Sodium tungstate (0.66 g) + oxalic acid (0.25 g)
-
Oxidant: 30 mL H₂O₂ (30%)
-
Temperature: 80°C
-
Time: 24 hours
Direct Oxidation of Hydroquinone
Traditional Oxidants: Chromium and Hypochlorite
Early methods employed chromium trioxide or sodium hypochlorite in acetone or acetic acid. While effective, these oxidants generate hazardous waste and require costly separation steps. For example, sodium hypochlorite oxidation in alkaline media yields 70–75% product but faces scalability challenges due to corrosive byproducts .
Hydrogen Peroxide-Based Oxidation
Modern approaches prioritize H₂O₂ for its eco-friendly profile. FeBr₃ or tungstate catalysts in water enable direct oxidation of hydroquinone at milder conditions (60–100°C, 6–12 hours), though yields remain lower (65–70%) compared to the two-step hydrogenation-oxidation route .
Catalytic Dehydrogenation
One-Step Hydrogenation-Oxidation
Patent DE19541262 describes a one-step process using palladium-carbon and sodium borate in diglyme under hydrogen. However, this method suffers from low selectivity (46.1%) and conversion (86.5%), limiting industrial adoption .
Key Limitations:
Gas-Phase Dehydrogenation
Emerging techniques explore gas-phase dehydrogenation of cyclohexanediols over metal oxide catalysts (e.g., CuO-ZnO/Al₂O₃) at 250–300°C. Preliminary data suggest yields up to 70%, but catalyst deactivation remains a hurdle .
Comparative Analysis of Methods
Method | Catalyst System | Solvent | Temperature (°C) | Yield (%) | Environmental Impact |
---|---|---|---|---|---|
H₂ Hydrogenation + H₂O₂ | Skeleton Ni → Na₂WO₄ | Water | 80–150 | 78–90 | Low (water-based) |
Direct H₂O₂ Oxidation | FeBr₃/Na₂WO₄ | Water | 60–100 | 65–70 | Low |
NaOCl Oxidation | Chromium catalysts | Acetone | 25–40 | 70–75 | High (toxic waste) |
One-Step Pd/C | Pd-C + Na₂B₄O₇ | Diglyme | 100–150 | <50 | Moderate |
Green Chemistry Innovations
Solvent-Free Systems
Recent patents highlight efforts to eliminate solvents entirely. For instance, melt-phase hydrogenation of hydroquinone at 160°C with Raney nickel achieves 88% diol yield, bypassing aqueous systems .
Catalyst Recycling
Skeleton nickel catalysts are reused for up to five cycles without significant activity loss, reducing costs by 30–40% .
Industrial Scalability and Challenges
While hydrogen peroxide-based methods dominate pilot-scale production, long reaction times (24–48 hours) and high catalyst loadings (5–10 wt%) necessitate further optimization. Advances in continuous-flow reactors and immobilized catalysts aim to address these issues .
Chemical Reactions Analysis
1,4-Cyclohexanedione undergoes various chemical reactions, including oxidation, reduction, and substitution reactions.
Reduction: The compound can be reduced to 1,4-cyclohexanediol using suitable reducing agents.
Substitution: It can undergo substitution reactions with various nucleophiles, leading to the formation of different substituted cyclohexanediones.
Common reagents used in these reactions include bromate, nitric acid, sulfuric acid, and reducing agents like sodium borohydride. Major products formed from these reactions include 1,4-dihydroxybenzene, 1,4-benzoquinone, and various substituted cyclohexanediones .
Scientific Research Applications
Pharmaceutical Applications
1,4-Cyclohexanedione is a crucial building block in the synthesis of various pharmaceuticals. Notably, it is involved in the production of analgesics such as Cebranopadol, which has shown promise for pain management due to its unique mechanism of action .
Case Study: Synthesis of Dihydroartemisinin
A recent study highlighted the use of this compound as a precursor for dihydroartemisinin, an antimalarial drug. The compound's hydroxyl groups facilitate functionalization reactions that lead to the production of bioactive derivatives .
Polymer Production
The compound serves as a monomer for synthesizing various polymers, including polycarbonates and polyurethanes. Its ability to undergo polymerization reactions makes it valuable in producing materials with desirable mechanical properties.
Table: Polymer Applications of this compound
Polymer Type | Application |
---|---|
Polycarbonates | Scratch-resistant coatings |
Polyurethanes | Flexible foams and elastomers |
Polyamides | High-strength fibers |
Agricultural Uses
In agriculture, this compound functions as a plant growth regulator. Its application can enhance crop yields by influencing plant metabolic processes .
Catalytic Processes
Recent advancements have demonstrated the potential of this compound in catalytic processes aimed at converting biomass into valuable chemicals. For instance, researchers have developed methods to transform lignin-derived compounds into cyclohexanediol and cyclohexanediamine using this diketone as an intermediate .
Case Study: Lignin Valorization
A study reported a two-step catalytic process where beech lignin-derived dimers were converted into 1,4-cyclohexanediol with an impressive yield of 86.5%. This approach not only enhances the value of lignin but also contributes to sustainable chemical production .
Chemical Research
This compound is extensively studied in chemical kinetics and oscillatory reactions. It has been used to investigate the bromate-1,4-cyclohexanedione-ferroin oscillating reaction system, providing insights into reaction mechanisms and dynamics .
Table: Research Applications
Research Area | Description |
---|---|
Kinetics | Study of oscillatory reactions |
Mechanistic Studies | Investigation of reaction pathways |
Material Science | Development of new materials from cyclohexanediol |
Mechanism of Action
The mechanism of action of 1,4-cyclohexanedione involves its ability to undergo various chemical reactions, such as oxidation and reduction. These reactions are facilitated by the presence of the two carbonyl groups in the cyclohexane ring, which can interact with different reagents and catalysts. The molecular targets and pathways involved depend on the specific reaction and application being studied .
Comparison with Similar Compounds
Comparison with Structurally Similar Compounds
1,2-Cyclohexanedione vs. 1,4-Cyclohexanedione
Key Insight : The para-diketone structure of this compound enables unique cyclization pathways unavailable to 1,2-isomers, making it valuable for complex heterocycles .
1,3-Cyclohexanedione vs. This compound
Key Insight: The 1,3-diketone’s higher enol content enhances its role in metal-mediated reactions, while this compound’s para-substitution favors sterically controlled cyclizations .
Cyclohexanone vs. This compound
Key Insight: The additional ketone in this compound increases its polarity and versatility in multi-step syntheses compared to cyclohexanone .
Derivatives: this compound Monoethylene Ketal
Key Insight : Ketal protection enhances stability for applications requiring controlled release or reduced side reactivity .
Substituted Derivatives: 2,2,6-Trimethyl-1,4-cyclohexanedione
Key Insight : Methyl substituents improve thermal stability and selectivity in syntheses requiring bulky intermediates .
Data Tables
Table 1: Physical Properties of this compound and Analogues
*Literature-estimated values.
Table 2: Catalytic Performance in Dehydrogenation
Catalyst | Temperature (°C) | Conversion (%) | Selectivity (%) | Reference |
---|---|---|---|---|
Pd/C | 300 | 47.7 | 90.0 (hydroquinone) | |
Cu-Ce-Co-Mg-Al | 300 | 47.7 | 90.0 (1,4-dione) |
Biological Activity
1,4-Cyclohexanedione (CHD) is a cyclic diketone that has garnered attention in various fields of biological research due to its unique structural properties and potential therapeutic applications. This article explores the biological activity of this compound, focusing on its synthesis, biological effects, and relevant case studies.
Chemical Structure and Properties
This compound is characterized by two carbonyl groups located at the 1 and 4 positions of a cyclohexane ring. Its molecular formula is , and it has a melting point of approximately 77-78.5 °C. The compound can exist in various tautomeric forms, influencing its reactivity and biological interactions.
Antimicrobial Properties
Research indicates that derivatives of cyclohexanedione exhibit significant antimicrobial activity. A study synthesized various metal complexes of cyclohexane-1,3-dione and assessed their antibacterial effects against several bacterial strains including Escherichia coli, Staphylococcus aureus, and Salmonella typhimurium. Some complexes demonstrated medium-level antibacterial activity compared to standard antibiotics like ampicillin .
Antidiabetic Potential
Cyclohexanedione derivatives have also been investigated for their potential in diabetes management. Compounds synthesized from cyclohexanedione have shown inhibitory effects on enzymes related to glucose metabolism, suggesting that they may serve as precursors for developing anti-diabetic medications .
Cytotoxicity and Cancer Research
In cancer research, studies have explored the cytotoxic effects of cyclohexanedione derivatives on various cancer cell lines. For instance, certain hydrazone derivatives derived from cyclohexanedione have been reported to exhibit anti-tumor activity against leukemia and sarcoma cells. These compounds may act through mechanisms involving apoptosis induction and cell cycle arrest .
Synthesis Methods
The synthesis of this compound can be achieved through several methods:
- Oxidation of Cyclohexene : Cyclohexene can be oxidized using potassium permanganate or other oxidizing agents to yield cyclohexanedione.
- Hydration of Cyclohexanone : Cyclohexanone can undergo hydration under acidic conditions to form this compound.
Case Study 1: Antibacterial Activity Assessment
A study conducted by researchers synthesized two new ligands derived from cyclohexane-1,3-dione and evaluated their antibacterial properties. The ligands were tested against clinical strains of bacteria, revealing that some metal complexes exhibited promising antibacterial activity, which could lead to the development of new antimicrobial agents .
Case Study 2: Anti-diabetic Drug Development
In another study focused on anti-diabetic drugs, researchers synthesized a series of hydrazone derivatives from this compound. These compounds were evaluated for their ability to inhibit α-glucosidase, an enzyme involved in carbohydrate digestion. The results indicated that some derivatives displayed significant inhibitory activity, suggesting their potential use in managing postprandial blood glucose levels .
Summary of Research Findings
Q & A
Basic Research Questions
Q. What are the key structural features of 1,4-cyclohexanedione, and how do they influence its reactivity?
- This compound (C₆H₈O₂) is a six-membered cyclic diketone with two ketone groups at the 1,4-positions. Its planar structure and electron-deficient carbonyl groups enable keto-enol tautomerism, which is critical in nucleophilic addition and condensation reactions. The molecule contains 16 bonds, including 8 non-H bonds, 2 double bonds, and a six-membered ring, as confirmed by quantum chemical calculations and spectral data . These features facilitate its use as a precursor in synthesizing heterocycles and spiro compounds.
Q. What are the standard methods for synthesizing this compound in high purity?
- A widely reported method involves the acid-catalyzed cyclization of diethyl succinate (123-25-1) under reflux conditions. Optimization includes using sodium ethoxide as a base and controlling reaction time (6–8 hours) to achieve yields >85% . Purification via recrystallization from ethanol ensures >98% purity, validated by melting point (76–78°C) and NMR spectroscopy .
Q. How can spectroscopic techniques (e.g., NMR, IR) distinguish this compound from its isomers?
- ¹H NMR : The molecule exhibits a singlet at δ 2.4–2.6 ppm for equivalent methylene protons adjacent to carbonyl groups.
- ¹³C NMR : Two carbonyl carbons resonate at δ 207–210 ppm.
- IR : Strong C=O stretching vibrations at 1715–1740 cm⁻¹, absent in non-ketonic isomers.
- These spectral markers are distinct from 1,3-cyclohexanedione, which shows split methylene signals and shifted carbonyl peaks .
Q. What solvents and conditions are optimal for recrystallizing this compound?
- Ethanol and methanol are preferred due to high solubility at elevated temperatures (50–60°C) and low solubility at room temperature. A solvent-to-product ratio of 5:1 (v/w) yields needle-like crystals with minimal impurities. Slow cooling (1–2°C/min) ensures uniform crystal growth .
Advanced Research Questions
Q. How does this compound participate in oscillating chemical reactions, and what mechanistic insights have been gained?
- In the bromate–this compound–ferroin system, the compound acts as a substrate in Belousov-Zhabotinsky (BZ)-type reactions. Its oxidation by bromate radicals generates intermediates (e.g., enol radicals), which drive periodic oscillations in redox potential. Studies show that ferroin concentrations >5 × 10⁻⁵ M are required to sustain oscillations, with spiral and transverse wave patterns observed in quasi-2D reaction-diffusion systems .
Q. What experimental parameters govern the continuous-flow dehydrogenation of this compound to hydroquinone?
- A structured multichannel reactor with 10 wt% Pd/C catalyst achieves >90% conversion at 120–150°C. Critical factors:
- Liquid flow rate : 0.5–1.0 mL/min to minimize pressure drop.
- Substrate concentration : 10–15 wt% in ethanol.
- Nitrogen flow : 50–100 mL/min to enhance mass transfer.
- Quadratic modeling (R² > 0.95) confirms temperature and substrate concentration as dominant variables for selectivity (75–85%) .
Q. How do tautomeric equilibria of this compound affect its reactivity in cross-aldol condensations?
- The enol form (≤5% abundance in DMSO-d₆) reacts preferentially with aldehydes via keto-enol tautomerism. DFT studies indicate a lower activation barrier (ΔG‡ ≈ 45 kJ/mol) for enol-mediated pathways compared to keto-form reactions (ΔG‡ > 60 kJ/mol). Acidic conditions (pH 4–5) stabilize the enolate, accelerating condensation .
Q. What strategies mitigate side reactions during the synthesis of this compound derivatives (e.g., dioximes or diamines)?
- For dioximes : Use hydroxylamine hydrochloride in aqueous ethanol (1:2 molar ratio) at 60°C. Monitor pH (6.5–7.0) to prevent overoxidation.
- For diamines : Catalytic hydrogenation (H₂, 50 psi) with Raney Ni at 80°C reduces dioximes selectively. Adding NH₄OH suppresses imine hydrolysis .
Q. How can this compound be integrated into spirocyclic frameworks, and what challenges arise?
- The compound reacts with endoperoxides (e.g., from cyclohexadienes) to form spiro-trioxanes. Key challenges:
- Regioselectivity : Steric hindrance at C2/C5 positions favors spiro-junction formation at C1/C4.
- Stability : Trioxanes require anhydrous conditions to prevent ring-opening.
- X-ray crystallography confirms chair conformations in spiro products .
Q. What contradictions exist in reported thermodynamic data for this compound, and how can they be resolved?
Properties
IUPAC Name |
cyclohexane-1,4-dione | |
---|---|---|
Source | PubChem | |
URL | https://pubchem.ncbi.nlm.nih.gov | |
Description | Data deposited in or computed by PubChem | |
InChI |
InChI=1S/C6H8O2/c7-5-1-2-6(8)4-3-5/h1-4H2 | |
Source | PubChem | |
URL | https://pubchem.ncbi.nlm.nih.gov | |
Description | Data deposited in or computed by PubChem | |
InChI Key |
DCZFGQYXRKMVFG-UHFFFAOYSA-N | |
Source | PubChem | |
URL | https://pubchem.ncbi.nlm.nih.gov | |
Description | Data deposited in or computed by PubChem | |
Canonical SMILES |
C1CC(=O)CCC1=O | |
Source | PubChem | |
URL | https://pubchem.ncbi.nlm.nih.gov | |
Description | Data deposited in or computed by PubChem | |
Molecular Formula |
C6H8O2 | |
Source | PubChem | |
URL | https://pubchem.ncbi.nlm.nih.gov | |
Description | Data deposited in or computed by PubChem | |
DSSTOX Substance ID |
DTXSID6060929 | |
Record name | 1,4-Cyclohexanedione | |
Source | EPA DSSTox | |
URL | https://comptox.epa.gov/dashboard/DTXSID6060929 | |
Description | DSSTox provides a high quality public chemistry resource for supporting improved predictive toxicology. | |
Molecular Weight |
112.13 g/mol | |
Source | PubChem | |
URL | https://pubchem.ncbi.nlm.nih.gov | |
Description | Data deposited in or computed by PubChem | |
Physical Description |
Yellow crystalline solid; [Aldrich MSDS] | |
Record name | 1,4-Cyclohexanedione | |
Source | Haz-Map, Information on Hazardous Chemicals and Occupational Diseases | |
URL | https://haz-map.com/Agents/9745 | |
Description | Haz-Map® is an occupational health database designed for health and safety professionals and for consumers seeking information about the adverse effects of workplace exposures to chemical and biological agents. | |
Explanation | Copyright (c) 2022 Haz-Map(R). All rights reserved. Unless otherwise indicated, all materials from Haz-Map are copyrighted by Haz-Map(R). No part of these materials, either text or image may be used for any purpose other than for personal use. Therefore, reproduction, modification, storage in a retrieval system or retransmission, in any form or by any means, electronic, mechanical or otherwise, for reasons other than personal use, is strictly prohibited without prior written permission. | |
CAS No. |
637-88-7 | |
Record name | 1,4-Cyclohexanedione | |
Source | CAS Common Chemistry | |
URL | https://commonchemistry.cas.org/detail?cas_rn=637-88-7 | |
Description | CAS Common Chemistry is an open community resource for accessing chemical information. Nearly 500,000 chemical substances from CAS REGISTRY cover areas of community interest, including common and frequently regulated chemicals, and those relevant to high school and undergraduate chemistry classes. This chemical information, curated by our expert scientists, is provided in alignment with our mission as a division of the American Chemical Society. | |
Explanation | The data from CAS Common Chemistry is provided under a CC-BY-NC 4.0 license, unless otherwise stated. | |
Record name | 1,4-Cyclohexanedione | |
Source | ChemIDplus | |
URL | https://pubchem.ncbi.nlm.nih.gov/substance/?source=chemidplus&sourceid=0000637887 | |
Description | ChemIDplus is a free, web search system that provides access to the structure and nomenclature authority files used for the identification of chemical substances cited in National Library of Medicine (NLM) databases, including the TOXNET system. | |
Record name | 1,4-Cyclohexanedione | |
Source | DTP/NCI | |
URL | https://dtp.cancer.gov/dtpstandard/servlet/dwindex?searchtype=NSC&outputformat=html&searchlist=7192 | |
Description | The NCI Development Therapeutics Program (DTP) provides services and resources to the academic and private-sector research communities worldwide to facilitate the discovery and development of new cancer therapeutic agents. | |
Explanation | Unless otherwise indicated, all text within NCI products is free of copyright and may be reused without our permission. Credit the National Cancer Institute as the source. | |
Record name | 1,4-Cyclohexanedione | |
Source | EPA Chemicals under the TSCA | |
URL | https://www.epa.gov/chemicals-under-tsca | |
Description | EPA Chemicals under the Toxic Substances Control Act (TSCA) collection contains information on chemicals and their regulations under TSCA, including non-confidential content from the TSCA Chemical Substance Inventory and Chemical Data Reporting. | |
Record name | 1,4-Cyclohexanedione | |
Source | EPA DSSTox | |
URL | https://comptox.epa.gov/dashboard/DTXSID6060929 | |
Description | DSSTox provides a high quality public chemistry resource for supporting improved predictive toxicology. | |
Record name | Cyclohexane-1,4-dione | |
Source | European Chemicals Agency (ECHA) | |
URL | https://echa.europa.eu/substance-information/-/substanceinfo/100.010.279 | |
Description | The European Chemicals Agency (ECHA) is an agency of the European Union which is the driving force among regulatory authorities in implementing the EU's groundbreaking chemicals legislation for the benefit of human health and the environment as well as for innovation and competitiveness. | |
Explanation | Use of the information, documents and data from the ECHA website is subject to the terms and conditions of this Legal Notice, and subject to other binding limitations provided for under applicable law, the information, documents and data made available on the ECHA website may be reproduced, distributed and/or used, totally or in part, for non-commercial purposes provided that ECHA is acknowledged as the source: "Source: European Chemicals Agency, http://echa.europa.eu/". Such acknowledgement must be included in each copy of the material. ECHA permits and encourages organisations and individuals to create links to the ECHA website under the following cumulative conditions: Links can only be made to webpages that provide a link to the Legal Notice page. | |
Record name | 1,4-CYCLOHEXANEDIONE | |
Source | FDA Global Substance Registration System (GSRS) | |
URL | https://gsrs.ncats.nih.gov/ginas/app/beta/substances/BJS27Z99AM | |
Description | The FDA Global Substance Registration System (GSRS) enables the efficient and accurate exchange of information on what substances are in regulated products. Instead of relying on names, which vary across regulatory domains, countries, and regions, the GSRS knowledge base makes it possible for substances to be defined by standardized, scientific descriptions. | |
Explanation | Unless otherwise noted, the contents of the FDA website (www.fda.gov), both text and graphics, are not copyrighted. They are in the public domain and may be republished, reprinted and otherwise used freely by anyone without the need to obtain permission from FDA. Credit to the U.S. Food and Drug Administration as the source is appreciated but not required. | |
Retrosynthesis Analysis
AI-Powered Synthesis Planning: Our tool employs the Template_relevance Pistachio, Template_relevance Bkms_metabolic, Template_relevance Pistachio_ringbreaker, Template_relevance Reaxys, Template_relevance Reaxys_biocatalysis model, leveraging a vast database of chemical reactions to predict feasible synthetic routes.
One-Step Synthesis Focus: Specifically designed for one-step synthesis, it provides concise and direct routes for your target compounds, streamlining the synthesis process.
Accurate Predictions: Utilizing the extensive PISTACHIO, BKMS_METABOLIC, PISTACHIO_RINGBREAKER, REAXYS, REAXYS_BIOCATALYSIS database, our tool offers high-accuracy predictions, reflecting the latest in chemical research and data.
Strategy Settings
Precursor scoring | Relevance Heuristic |
---|---|
Min. plausibility | 0.01 |
Model | Template_relevance |
Template Set | Pistachio/Bkms_metabolic/Pistachio_ringbreaker/Reaxys/Reaxys_biocatalysis |
Top-N result to add to graph | 6 |
Feasible Synthetic Routes
Disclaimer and Information on In-Vitro Research Products
Please be aware that all articles and product information presented on BenchChem are intended solely for informational purposes. The products available for purchase on BenchChem are specifically designed for in-vitro studies, which are conducted outside of living organisms. In-vitro studies, derived from the Latin term "in glass," involve experiments performed in controlled laboratory settings using cells or tissues. It is important to note that these products are not categorized as medicines or drugs, and they have not received approval from the FDA for the prevention, treatment, or cure of any medical condition, ailment, or disease. We must emphasize that any form of bodily introduction of these products into humans or animals is strictly prohibited by law. It is essential to adhere to these guidelines to ensure compliance with legal and ethical standards in research and experimentation.