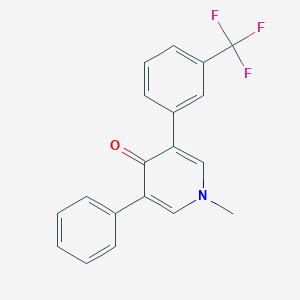
Fluridone
Overview
Description
Fluridone is an organic compound widely used as an aquatic herbicide to control invasive plants such as hydrilla and Eurasian watermilfoil . It is a colorless solid with the chemical formula C₁₉H₁₄F₃NO and a molar mass of 329.3 g/mol . This compound was first reported as a potential herbicide for cotton fields in 1976 and was registered with the United States Environmental Protection Agency in 1986 .
Mechanism of Action
Target of Action
Fluridone primarily targets the enzyme Phytoene Desaturase (PDS) . PDS is a crucial enzyme in the carotenoid biosynthetic pathway, which is responsible for the conversion of phytoene to colored carotenoids .
Mode of Action
This compound is a systemic herbicide that works by inhibiting the function of PDS . This inhibition disrupts the carotenoid biosynthetic pathway, leading to the degradation or “bleaching” of chlorophyll by sunlight . The absence of carotenoids causes degradation of chlorophyll, leading to the whitening or bleaching of plants .
Biochemical Pathways
This compound affects the carotenoid biosynthetic pathway by inhibiting the function of PDS . This disruption leads to a decrease in the production of carotenoids, which are pigments that protect chlorophyll molecules from photodegradation . As a result, chlorophyll degrades, leading to the bleaching of plants .
Pharmacokinetics
This compound is absorbed and excreted in the feces within 72 hours of oral administration to rats . In the environment, this compound breaks down over days or weeks, with the major degradation product being N-methyl formamide . It is stable to oxidation and hydrolysis, and its volatilization is insignificant . The breakdown of this compound in the aquatic environment occurs mostly through photolysis .
Result of Action
The inhibition of PDS by this compound leads to a decrease in the production of carotenoids, resulting in the degradation of chlorophyll . This causes the plants to become whitish-pink or chlorotic at growing points and die slowly . In addition, this compound can affect the accumulation of astaxanthin and growth of Haematococcus pluvialis by regulating the synthesis of carotenoids, chlorophyll, and fatty acids .
Action Environment
This compound is used to manage aquatic vegetation in fresh water ponds, lakes, reservoirs, canals, and rivers . Its effectiveness depends on the degree to which the herbicide maintains contact with plants . Rapid water movement or any dilution of this herbicide in water will reduce its effectiveness . This compound concentrations in the water were generally below the 2–5 parts per billion required for SAV control . Monitoring demonstrated that these low water concentrations were likely due to dissipation by tides, despite the use of pelleted this compound formulations marketed for flowing water environments .
Biochemical Analysis
Biochemical Properties
Fluridone interacts with the enzyme phytoene desaturase, a rate-limiting enzyme that catalyzes the conversion of phytoene to colored carotenoids . By inhibiting this enzyme, this compound disrupts the carotenoid biosynthetic pathway . This interaction is crucial for this compound’s role as a herbicide, as it leads to the degradation of chlorophyll and the bleaching of leaves .
Cellular Effects
This compound has significant effects on various types of cells and cellular processes. In rice seedlings, this compound treatment resulted in leaf bleaching by decreasing pigment content . It significantly downregulated the transcription levels of leaf pigment biosynthetic and catabolic genes . In Haematococcus pluvialis, a microalga known for its high astaxanthin-producing ability, this compound significantly inhibited growth and accumulation of astaxanthin .
Molecular Mechanism
This compound exerts its effects at the molecular level primarily by inhibiting the enzyme phytoene desaturase . This inhibition disrupts the carotenoid biosynthetic pathway, leading to a decrease in carotene formation and subsequent chlorophyll degradation . In addition, this compound has been found to downregulate ABA biosynthesis genes and upregulate an ABA catabolic gene, contributing to reduced ABA levels .
Temporal Effects in Laboratory Settings
This compound’s effects change over time in laboratory settings. For instance, in a study conducted in the Sacramento-San Joaquin Delta, this compound concentrations in the water were generally below the required levels for SAV control, likely due to dissipation by tides . This compound did accumulate in sediment at concentrations hundreds of times higher than those measured in the water .
Dosage Effects in Animal Models
One study found that fishes’ livers were enlarged and their ability to catch prey decreased significantly when exposed to this compound .
Metabolic Pathways
This compound is involved in the carotenoid biosynthetic pathway . It inhibits the enzyme phytoene desaturase, disrupting the conversion of phytoene to colored carotenoids . This disruption leads to a decrease in carotene formation and subsequent chlorophyll degradation .
Transport and Distribution
This compound’s transport and distribution within cells and tissues are influenced by its strong sorbance to organic matter . In the Sacramento-San Joaquin Delta study, this compound was found to accumulate in sediment at concentrations hundreds of times higher than those measured in the water .
Subcellular Localization
Given its role as a herbicide and its mechanism of action, it can be inferred that this compound likely localizes to the chloroplasts where it can interfere with the carotenoid biosynthetic pathway .
Preparation Methods
Synthetic Routes and Reaction Conditions
Fluridone can be synthesized through a multi-step process involving the reaction of 3-(trifluoromethyl)benzaldehyde with acetophenone in the presence of a base to form 1-(3-(trifluoromethyl)phenyl)-3-phenylprop-2-en-1-one. This intermediate is then cyclized using ammonium acetate to yield this compound .
Industrial Production Methods
In industrial settings, this compound is produced using similar synthetic routes but optimized for large-scale production. The reaction conditions are carefully controlled to ensure high yield and purity of the final product. The compound is available in both liquid formulations and slow-release solid forms .
Chemical Reactions Analysis
Types of Reactions
Fluridone undergoes various chemical reactions, including:
Oxidation: This compound can be oxidized to form N-methyl formamide as a major degradation product.
Reduction: Reduction reactions involving this compound are less common but can occur under specific conditions.
Substitution: this compound can undergo substitution reactions, particularly in the presence of strong nucleophiles.
Common Reagents and Conditions
Oxidation: Common oxidizing agents such as hydrogen peroxide or potassium permanganate can be used.
Reduction: Reducing agents like sodium borohydride may be employed under controlled conditions.
Substitution: Strong nucleophiles such as sodium methoxide can facilitate substitution reactions.
Major Products Formed
Oxidation: N-methyl formamide is a significant product of this compound oxidation.
Reduction: Reduced forms of this compound, though less common, can be synthesized.
Substitution: Substituted derivatives of this compound can be obtained depending on the nucleophile used.
Scientific Research Applications
Aquatic Herbicide
Mechanism of Action:
Fluridone inhibits phytoene desaturase, an enzyme critical for carotenoid biosynthesis in plants. This inhibition disrupts photosynthesis, leading to plant death over time. It is effective against species like Eurasian watermilfoil and hydrilla, which are notorious for their invasiveness in freshwater ecosystems.
Case Study: Efficacy in Lakes
A study published in "Aquatic Toxicology" showed that this compound requires multiple applications over extended periods (45 to 90 days) to effectively manage invasive aquatic plants. The research indicated that while this compound did not significantly affect fish survivorship, it posed risks related to prey capture and endocrine disruption in species such as fathead minnows (Pimephales promelas) at certain concentrations .
Table 1: Effects of this compound on Aquatic Species
Species | Concentration (mg/L) | Observed Effects |
---|---|---|
Fathead Minnow | 0.2 - 0.6 | No significant growth impact; endocrine disruption noted |
Channel Catfish | ≤0.5 | No negative effects on growth or survival |
Daphnids | ≤0.2 | No significant mortality observed |
Agricultural Applications
Seed Germination Stimulation:
Research has shown that this compound can serve as a germination stimulant for certain crops by breaking seed dormancy. A study demonstrated that applying this compound to dormant seeds of Lolium rigidum resulted in stimulated germination followed by high seedling mortality, suggesting its potential as a dual-purpose herbicide and germination enhancer .
Table 2: Germination Effects of this compound on Various Seeds
Seed Type | Treatment Conditions | Germination Rate (%) | Seedling Survival (%) |
---|---|---|---|
Lolium rigidum | Low organic matter soil | High | Low |
Wheat | This compound-treated sandy loam | Moderate | Bleached |
Lupins | This compound-treated sandy loam | Normal | Normal |
Environmental Impact Studies
Persistence and Degradation:
this compound’s persistence in aquatic environments has been a subject of extensive research. A study indicated that this compound has a half-life exceeding 150 days in natural lake conditions, highlighting its long-term presence and potential ecological impacts . Laboratory studies showed its susceptibility to photodegradation and microbial degradation, but these processes were less effective than previously assumed in real-world scenarios.
Table 3: Persistence of this compound in Aquatic Environments
Environment | Half-Life (Days) | Dominant Degradation Pathway |
---|---|---|
Laboratory Conditions | 2.3 - 118 | Photodegradation |
Natural Lakes | >150 | Microbial degradation (57 days) |
Regulatory Considerations
The Environmental Protection Agency (EPA) mandates rigorous testing of this compound's effects on non-target species before commercial use. Although initial studies indicate low toxicity at high concentrations, concerns remain regarding the effects at lower concentrations typically found during herbicide application .
Comparison with Similar Compounds
Similar Compounds
Norflurazon: Another herbicide that inhibits phytoene desaturase and disrupts carotenoid biosynthesis.
Sulcotrione: Inhibits 4-hydroxyphenylpyruvate dioxygenase, affecting chlorophyll and carotenoid synthesis.
Uniqueness of Fluridone
This compound is unique in its strong binding to organic matter in sediments, limiting its transport through soil and water . This property makes it particularly effective in controlling invasive aquatic plants in various environments .
Biological Activity
Fluridone is a synthetic herbicide primarily used for the control of aquatic weeds, particularly Hydrilla verticillata and Eurasian watermilfoil. Its mode of action involves the inhibition of carotenoid synthesis, leading to impaired photosynthesis and eventual plant death. This article explores the biological activity of this compound, detailing its effects on various organisms, its biochemical mechanisms, and relevant case studies.
This compound functions as a phytoene desaturase inhibitor , disrupting the biosynthesis of carotenoids, which are essential for photosynthesis and photoprotection in plants. By inhibiting this pathway, this compound causes a reduction in chlorophyll levels and increases susceptibility to photooxidative damage. The specific biochemical pathways affected include:
- Carotenoid Biosynthesis : this compound inhibits the expression of genes involved in carotenoid synthesis, leading to decreased astaxanthin accumulation in Haematococcus pluvialis and other algae .
- Photosynthesis : The reduction in carotenoids negatively impacts the photosynthetic efficiency of treated plants, as evidenced by decreased chlorophyll content .
- Fatty Acid Metabolism : this compound treatment alters fatty acid metabolism, with varying effects depending on the developmental stage of the organism .
1. Impact on Algae
Research has shown that this compound significantly inhibits growth and astaxanthin accumulation in Haematococcus pluvialis. In a study utilizing transcriptome sequencing, it was found that this compound treatment led to downregulation of key genes associated with photosynthesis and carotenoid synthesis while upregulating certain antioxidant genes. This indicates a complex response where algae attempt to mitigate oxidative stress caused by reduced carotenoid levels .
2. Fish Health
This compound has been studied for its effects on fish health. A notable study involving fathead minnows (Pimephales promelas) revealed that while this compound did not significantly affect fish survivorship or growth, it did impact more subtle health indicators such as prey capture ability and endocrine function. Adult males exhibited increased nuptial tubercles, suggesting potential endocrine disruption linked to altered hormone levels due to this compound exposure . Additionally, liver enlargement was observed, which may indicate stress responses or metabolic alterations related to this compound exposure .
Case Study 1: Hydrilla Management
In a management project conducted in Florida, this compound was applied to control Hydrilla verticillata over a large area. The study monitored hydrosoil residues and plant biomass over several months. Results indicated that while this compound effectively reduced hydrilla biomass (up to 90% reduction), it did not adversely affect non-target submerged vegetation during treatment periods . This case highlights this compound's efficacy as an aquatic herbicide while raising considerations for ecosystem balance.
Case Study 2: Resistance Development
A population genetics study identified the emergence of this compound-resistant Hydrilla populations in Florida. Molecular techniques confirmed resistance traits linked to specific genetic mutations. The study suggested that continuous application of this compound could lead to increased resistance over time, necessitating integrated management strategies to mitigate this risk .
Data Tables
Properties
IUPAC Name |
1-methyl-3-phenyl-5-[3-(trifluoromethyl)phenyl]pyridin-4-one | |
---|---|---|
Source | PubChem | |
URL | https://pubchem.ncbi.nlm.nih.gov | |
Description | Data deposited in or computed by PubChem | |
InChI |
InChI=1S/C19H14F3NO/c1-23-11-16(13-6-3-2-4-7-13)18(24)17(12-23)14-8-5-9-15(10-14)19(20,21)22/h2-12H,1H3 | |
Source | PubChem | |
URL | https://pubchem.ncbi.nlm.nih.gov | |
Description | Data deposited in or computed by PubChem | |
InChI Key |
YWBVHLJPRPCRSD-UHFFFAOYSA-N | |
Source | PubChem | |
URL | https://pubchem.ncbi.nlm.nih.gov | |
Description | Data deposited in or computed by PubChem | |
Canonical SMILES |
CN1C=C(C(=O)C(=C1)C2=CC(=CC=C2)C(F)(F)F)C3=CC=CC=C3 | |
Source | PubChem | |
URL | https://pubchem.ncbi.nlm.nih.gov | |
Description | Data deposited in or computed by PubChem | |
Molecular Formula |
C19H14F3NO | |
Source | PubChem | |
URL | https://pubchem.ncbi.nlm.nih.gov | |
Description | Data deposited in or computed by PubChem | |
DSSTOX Substance ID |
DTXSID8024107 | |
Record name | 1-Methyl-3-phenyl-5-(3-(trifluoromethyl)phenyl)-4-pyridone | |
Source | EPA DSSTox | |
URL | https://comptox.epa.gov/dashboard/DTXSID8024107 | |
Description | DSSTox provides a high quality public chemistry resource for supporting improved predictive toxicology. | |
Molecular Weight |
329.3 g/mol | |
Source | PubChem | |
URL | https://pubchem.ncbi.nlm.nih.gov | |
Description | Data deposited in or computed by PubChem | |
Physical Description |
White solid; [HSDB] Colorless solid; [MSDSonline] | |
Record name | Fluridone | |
Source | Haz-Map, Information on Hazardous Chemicals and Occupational Diseases | |
URL | https://haz-map.com/Agents/5359 | |
Description | Haz-Map® is an occupational health database designed for health and safety professionals and for consumers seeking information about the adverse effects of workplace exposures to chemical and biological agents. | |
Explanation | Copyright (c) 2022 Haz-Map(R). All rights reserved. Unless otherwise indicated, all materials from Haz-Map are copyrighted by Haz-Map(R). No part of these materials, either text or image may be used for any purpose other than for personal use. Therefore, reproduction, modification, storage in a retrieval system or retransmission, in any form or by any means, electronic, mechanical or otherwise, for reasons other than personal use, is strictly prohibited without prior written permission. | |
Solubility |
Solubility in methanol, chloroform, diethyl ether: >10 g/L; in ethyl acetate: >5 g/L; in hexane: <0.5 g/L, In water, 12 mg/L at pH 7, 25 °C | |
Record name | FLURIDONE | |
Source | Hazardous Substances Data Bank (HSDB) | |
URL | https://pubchem.ncbi.nlm.nih.gov/source/hsdb/6653 | |
Description | The Hazardous Substances Data Bank (HSDB) is a toxicology database that focuses on the toxicology of potentially hazardous chemicals. It provides information on human exposure, industrial hygiene, emergency handling procedures, environmental fate, regulatory requirements, nanomaterials, and related areas. The information in HSDB has been assessed by a Scientific Review Panel. | |
Density |
Bulk density, loose: 0.358 g/cu cm; packed: 0.515 g/cu cm | |
Record name | FLURIDONE | |
Source | Hazardous Substances Data Bank (HSDB) | |
URL | https://pubchem.ncbi.nlm.nih.gov/source/hsdb/6653 | |
Description | The Hazardous Substances Data Bank (HSDB) is a toxicology database that focuses on the toxicology of potentially hazardous chemicals. It provides information on human exposure, industrial hygiene, emergency handling procedures, environmental fate, regulatory requirements, nanomaterials, and related areas. The information in HSDB has been assessed by a Scientific Review Panel. | |
Vapor Pressure |
0.0000001 [mmHg], 0.013 mPa (9.8X10-8 mm Hg) at 25 °C | |
Record name | Fluridone | |
Source | Haz-Map, Information on Hazardous Chemicals and Occupational Diseases | |
URL | https://haz-map.com/Agents/5359 | |
Description | Haz-Map® is an occupational health database designed for health and safety professionals and for consumers seeking information about the adverse effects of workplace exposures to chemical and biological agents. | |
Explanation | Copyright (c) 2022 Haz-Map(R). All rights reserved. Unless otherwise indicated, all materials from Haz-Map are copyrighted by Haz-Map(R). No part of these materials, either text or image may be used for any purpose other than for personal use. Therefore, reproduction, modification, storage in a retrieval system or retransmission, in any form or by any means, electronic, mechanical or otherwise, for reasons other than personal use, is strictly prohibited without prior written permission. | |
Record name | FLURIDONE | |
Source | Hazardous Substances Data Bank (HSDB) | |
URL | https://pubchem.ncbi.nlm.nih.gov/source/hsdb/6653 | |
Description | The Hazardous Substances Data Bank (HSDB) is a toxicology database that focuses on the toxicology of potentially hazardous chemicals. It provides information on human exposure, industrial hygiene, emergency handling procedures, environmental fate, regulatory requirements, nanomaterials, and related areas. The information in HSDB has been assessed by a Scientific Review Panel. | |
Color/Form |
White crystalline solid | |
CAS No. |
59756-60-4 | |
Record name | Fluridone | |
Source | CAS Common Chemistry | |
URL | https://commonchemistry.cas.org/detail?cas_rn=59756-60-4 | |
Description | CAS Common Chemistry is an open community resource for accessing chemical information. Nearly 500,000 chemical substances from CAS REGISTRY cover areas of community interest, including common and frequently regulated chemicals, and those relevant to high school and undergraduate chemistry classes. This chemical information, curated by our expert scientists, is provided in alignment with our mission as a division of the American Chemical Society. | |
Explanation | The data from CAS Common Chemistry is provided under a CC-BY-NC 4.0 license, unless otherwise stated. | |
Record name | Fluridone [ANSI:BSI:ISO] | |
Source | ChemIDplus | |
URL | https://pubchem.ncbi.nlm.nih.gov/substance/?source=chemidplus&sourceid=0059756604 | |
Description | ChemIDplus is a free, web search system that provides access to the structure and nomenclature authority files used for the identification of chemical substances cited in National Library of Medicine (NLM) databases, including the TOXNET system. | |
Record name | 4(1H)-Pyridinone, 1-methyl-3-phenyl-5-[3-(trifluoromethyl)phenyl]- | |
Source | EPA Chemicals under the TSCA | |
URL | https://www.epa.gov/chemicals-under-tsca | |
Description | EPA Chemicals under the Toxic Substances Control Act (TSCA) collection contains information on chemicals and their regulations under TSCA, including non-confidential content from the TSCA Chemical Substance Inventory and Chemical Data Reporting. | |
Record name | 1-Methyl-3-phenyl-5-(3-(trifluoromethyl)phenyl)-4-pyridone | |
Source | EPA DSSTox | |
URL | https://comptox.epa.gov/dashboard/DTXSID8024107 | |
Description | DSSTox provides a high quality public chemistry resource for supporting improved predictive toxicology. | |
Record name | 1-methyl-3-phenyl-5-[3-(trifluoromethyl)phenyl]-4-pyridone | |
Source | European Chemicals Agency (ECHA) | |
URL | https://echa.europa.eu/substance-information/-/substanceinfo/100.056.269 | |
Description | The European Chemicals Agency (ECHA) is an agency of the European Union which is the driving force among regulatory authorities in implementing the EU's groundbreaking chemicals legislation for the benefit of human health and the environment as well as for innovation and competitiveness. | |
Explanation | Use of the information, documents and data from the ECHA website is subject to the terms and conditions of this Legal Notice, and subject to other binding limitations provided for under applicable law, the information, documents and data made available on the ECHA website may be reproduced, distributed and/or used, totally or in part, for non-commercial purposes provided that ECHA is acknowledged as the source: "Source: European Chemicals Agency, http://echa.europa.eu/". Such acknowledgement must be included in each copy of the material. ECHA permits and encourages organisations and individuals to create links to the ECHA website under the following cumulative conditions: Links can only be made to webpages that provide a link to the Legal Notice page. | |
Record name | FLURIDONE | |
Source | FDA Global Substance Registration System (GSRS) | |
URL | https://gsrs.ncats.nih.gov/ginas/app/beta/substances/3L0JQA61JX | |
Description | The FDA Global Substance Registration System (GSRS) enables the efficient and accurate exchange of information on what substances are in regulated products. Instead of relying on names, which vary across regulatory domains, countries, and regions, the GSRS knowledge base makes it possible for substances to be defined by standardized, scientific descriptions. | |
Explanation | Unless otherwise noted, the contents of the FDA website (www.fda.gov), both text and graphics, are not copyrighted. They are in the public domain and may be republished, reprinted and otherwise used freely by anyone without the need to obtain permission from FDA. Credit to the U.S. Food and Drug Administration as the source is appreciated but not required. | |
Record name | FLURIDONE | |
Source | Hazardous Substances Data Bank (HSDB) | |
URL | https://pubchem.ncbi.nlm.nih.gov/source/hsdb/6653 | |
Description | The Hazardous Substances Data Bank (HSDB) is a toxicology database that focuses on the toxicology of potentially hazardous chemicals. It provides information on human exposure, industrial hygiene, emergency handling procedures, environmental fate, regulatory requirements, nanomaterials, and related areas. The information in HSDB has been assessed by a Scientific Review Panel. | |
Melting Point |
154-155 °C | |
Record name | FLURIDONE | |
Source | Hazardous Substances Data Bank (HSDB) | |
URL | https://pubchem.ncbi.nlm.nih.gov/source/hsdb/6653 | |
Description | The Hazardous Substances Data Bank (HSDB) is a toxicology database that focuses on the toxicology of potentially hazardous chemicals. It provides information on human exposure, industrial hygiene, emergency handling procedures, environmental fate, regulatory requirements, nanomaterials, and related areas. The information in HSDB has been assessed by a Scientific Review Panel. | |
Retrosynthesis Analysis
AI-Powered Synthesis Planning: Our tool employs the Template_relevance Pistachio, Template_relevance Bkms_metabolic, Template_relevance Pistachio_ringbreaker, Template_relevance Reaxys, Template_relevance Reaxys_biocatalysis model, leveraging a vast database of chemical reactions to predict feasible synthetic routes.
One-Step Synthesis Focus: Specifically designed for one-step synthesis, it provides concise and direct routes for your target compounds, streamlining the synthesis process.
Accurate Predictions: Utilizing the extensive PISTACHIO, BKMS_METABOLIC, PISTACHIO_RINGBREAKER, REAXYS, REAXYS_BIOCATALYSIS database, our tool offers high-accuracy predictions, reflecting the latest in chemical research and data.
Strategy Settings
Precursor scoring | Relevance Heuristic |
---|---|
Min. plausibility | 0.01 |
Model | Template_relevance |
Template Set | Pistachio/Bkms_metabolic/Pistachio_ringbreaker/Reaxys/Reaxys_biocatalysis |
Top-N result to add to graph | 6 |
Feasible Synthetic Routes
Disclaimer and Information on In-Vitro Research Products
Please be aware that all articles and product information presented on BenchChem are intended solely for informational purposes. The products available for purchase on BenchChem are specifically designed for in-vitro studies, which are conducted outside of living organisms. In-vitro studies, derived from the Latin term "in glass," involve experiments performed in controlled laboratory settings using cells or tissues. It is important to note that these products are not categorized as medicines or drugs, and they have not received approval from the FDA for the prevention, treatment, or cure of any medical condition, ailment, or disease. We must emphasize that any form of bodily introduction of these products into humans or animals is strictly prohibited by law. It is essential to adhere to these guidelines to ensure compliance with legal and ethical standards in research and experimentation.