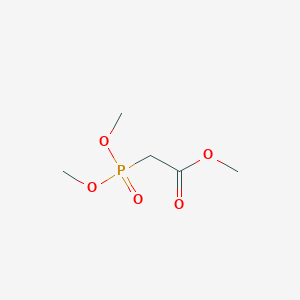
Trimethyl phosphonoacetate
Overview
Description
Trimethyl phosphonoacetate, also known as this compound, is a useful research compound. Its molecular formula is C5H11O5P and its molecular weight is 182.11 g/mol. The purity is usually 95%.
The exact mass of the compound this compound is unknown and the complexity rating of the compound is unknown. The compound has been submitted to the National Cancer Institute (NCI) for testing and evaluation and the Cancer Chemotherapy National Service Center (NSC) number is 84262. Its Medical Subject Headings (MeSH) category is Chemicals and Drugs Category - Organic Chemicals - Organophosphorus Compounds - Organophosphates - Supplementary Records. The United Nations designated GHS hazard class pictogram is Irritant, and the GHS signal word is WarningThe storage condition is unknown. Please store according to label instructions upon receipt of goods.
BenchChem offers high-quality this compound suitable for many research applications. Different packaging options are available to accommodate customers' requirements. Please inquire for more information about this compound including the price, delivery time, and more detailed information at info@benchchem.com.
Scientific Research Applications
Chemical Reactions and Synthesis : TPFA has been used in chemical synthesis, particularly in reactions involving aromatic ketophosphonates. For instance, Trimethyl phosphite can react with aromatic ketophosphonates in the presence of proton donors, leading to reverse nucleophilic addition to carbonyl groups (Griffiths, Jamali, & Tebby, 1985). Additionally, TPFA is used in the synthesis of β-keto phosphonates, a method suitable for both laboratory and large-scale production (Kim, Kong, & Lee, 1997).
Antiviral Research : TPFA and related compounds have shown potential as inhibitors of viral nucleic acid polymerases. For example, TPFA selectively inhibits HIV-1 reverse transcriptase and other herpesvirus DNA polymerases (McKenna et al., 1990).
Drug Delivery : Phosphonoacetate-trilactoside conjugate, a derivative of TPFA, shows potential for targeted drug delivery to the human liver, offering a yield of 23% and a short synthesis time (Bhat, Vaino, & Szarek, 2000).
Therapeutic Applications : TPFA and its derivatives have been explored for therapeutic applications. For instance, Phosphonoacetic acid, a related compound, has been investigated for its antiviral properties, particularly in the treatment of herpesvirus infections (Overby, Duff, & Mao, 1977).
Material Science : In the field of material science, high ion content trimethylphosphonium polystyrenes, which are related to TPFA, have demonstrated a significant increase in glass transition temperature, possibly due to ion clustering (Stokes, Orlicki, & Beyer, 2011).
Cancer Research : Phosphonoacetic acid, closely related to TPFA, shows promise as a potential cancer drug, with well-tolerated doses and effective results in preclinical trials (Roboz, Suzuki, Bekesi, & Hunt, 1977).
Computational Chemistry : TPFA has been studied in computational chemistry, particularly in the Horner-Wadsworth-Emmons reaction, which involves the addition of lithium enolate to acetaldehyde (Ando, 1999).
Mechanism of Action
Target of Action
Trimethyl phosphonoacetate is primarily used as a reactant in organic synthesis . Its primary targets are various organic compounds, particularly carbonyl compounds, with which it interacts to form phosphonate intermediates .
Mode of Action
This compound interacts with its targets through several types of reactions. These include intramolecular Mannich-type reactions, organocatalytic oxa-Michael reactions, prenylation and geranylation of oxindoles, intramolecular Horner-Wadsworth-Emmons reactions, and olefin cross-metathesis/heterocyclization . These reactions result in the formation of various organic compounds, including the sarain A diazatricyclic core .
Biochemical Pathways
The biochemical pathways affected by this compound are those involved in the synthesis of various organic compounds. The compound’s action can lead to the formation of new carbon-carbon bonds, enabling the synthesis of complex organic molecules .
Pharmacokinetics
Its physical properties, such as its boiling point (118 °c at 085 mmHg) and density (1125 g/mL at 25 °C), may influence its behavior in chemical reactions .
Result of Action
The result of this compound’s action is the formation of various organic compounds through the reactions mentioned above . These compounds can have a wide range of applications, from pharmaceuticals to materials science.
Biochemical Analysis
Biochemical Properties
Trimethyl phosphonoacetate participates in several biochemical reactions. It reacts with carbonyl compounds to form phosphonate intermediates, which are involved in a series of organic synthesis reactions . It is also used in the synthesis of scaffold compounds and in regulating the distribution and metabolic reactions of certain compounds .
Molecular Mechanism
This compound is involved in intramolecular Mannich-type reactions to produce the sarain A diazatricyclic core, organocatalytic oxa-Michael reactions, prenylation and geranylation of oxindoles, intramolecular Horner-Wadsworth-Emmons reactions, and olefin cross-metathesis/heterocyclization .
Temporal Effects in Laboratory Settings
Properties
IUPAC Name |
methyl 2-dimethoxyphosphorylacetate | |
---|---|---|
Source | PubChem | |
URL | https://pubchem.ncbi.nlm.nih.gov | |
Description | Data deposited in or computed by PubChem | |
InChI |
InChI=1S/C5H11O5P/c1-8-5(6)4-11(7,9-2)10-3/h4H2,1-3H3 | |
Source | PubChem | |
URL | https://pubchem.ncbi.nlm.nih.gov | |
Description | Data deposited in or computed by PubChem | |
InChI Key |
SIGOIUCRXKUEIG-UHFFFAOYSA-N | |
Source | PubChem | |
URL | https://pubchem.ncbi.nlm.nih.gov | |
Description | Data deposited in or computed by PubChem | |
Canonical SMILES |
COC(=O)CP(=O)(OC)OC | |
Source | PubChem | |
URL | https://pubchem.ncbi.nlm.nih.gov | |
Description | Data deposited in or computed by PubChem | |
Molecular Formula |
C5H11O5P | |
Source | PubChem | |
URL | https://pubchem.ncbi.nlm.nih.gov | |
Description | Data deposited in or computed by PubChem | |
DSSTOX Substance ID |
DTXSID3064063 | |
Record name | Acetic acid, (dimethoxyphosphinyl)-, methyl ester | |
Source | EPA DSSTox | |
URL | https://comptox.epa.gov/dashboard/DTXSID3064063 | |
Description | DSSTox provides a high quality public chemistry resource for supporting improved predictive toxicology. | |
Molecular Weight |
182.11 g/mol | |
Source | PubChem | |
URL | https://pubchem.ncbi.nlm.nih.gov | |
Description | Data deposited in or computed by PubChem | |
CAS No. |
5927-18-4 | |
Record name | Trimethyl phosphonoacetate | |
Source | CAS Common Chemistry | |
URL | https://commonchemistry.cas.org/detail?cas_rn=5927-18-4 | |
Description | CAS Common Chemistry is an open community resource for accessing chemical information. Nearly 500,000 chemical substances from CAS REGISTRY cover areas of community interest, including common and frequently regulated chemicals, and those relevant to high school and undergraduate chemistry classes. This chemical information, curated by our expert scientists, is provided in alignment with our mission as a division of the American Chemical Society. | |
Explanation | The data from CAS Common Chemistry is provided under a CC-BY-NC 4.0 license, unless otherwise stated. | |
Record name | Trimethylacetyl phosphate | |
Source | ChemIDplus | |
URL | https://pubchem.ncbi.nlm.nih.gov/substance/?source=chemidplus&sourceid=0005927184 | |
Description | ChemIDplus is a free, web search system that provides access to the structure and nomenclature authority files used for the identification of chemical substances cited in National Library of Medicine (NLM) databases, including the TOXNET system. | |
Record name | 5927-18-4 | |
Source | DTP/NCI | |
URL | https://dtp.cancer.gov/dtpstandard/servlet/dwindex?searchtype=NSC&outputformat=html&searchlist=84262 | |
Description | The NCI Development Therapeutics Program (DTP) provides services and resources to the academic and private-sector research communities worldwide to facilitate the discovery and development of new cancer therapeutic agents. | |
Explanation | Unless otherwise indicated, all text within NCI products is free of copyright and may be reused without our permission. Credit the National Cancer Institute as the source. | |
Record name | Acetic acid, 2-(dimethoxyphosphinyl)-, methyl ester | |
Source | EPA Chemicals under the TSCA | |
URL | https://www.epa.gov/chemicals-under-tsca | |
Description | EPA Chemicals under the Toxic Substances Control Act (TSCA) collection contains information on chemicals and their regulations under TSCA, including non-confidential content from the TSCA Chemical Substance Inventory and Chemical Data Reporting. | |
Record name | Acetic acid, (dimethoxyphosphinyl)-, methyl ester | |
Source | EPA DSSTox | |
URL | https://comptox.epa.gov/dashboard/DTXSID3064063 | |
Description | DSSTox provides a high quality public chemistry resource for supporting improved predictive toxicology. | |
Record name | Trimethyl phosphonoacetate | |
Source | European Chemicals Agency (ECHA) | |
URL | https://echa.europa.eu/substance-information/-/substanceinfo/100.025.148 | |
Description | The European Chemicals Agency (ECHA) is an agency of the European Union which is the driving force among regulatory authorities in implementing the EU's groundbreaking chemicals legislation for the benefit of human health and the environment as well as for innovation and competitiveness. | |
Explanation | Use of the information, documents and data from the ECHA website is subject to the terms and conditions of this Legal Notice, and subject to other binding limitations provided for under applicable law, the information, documents and data made available on the ECHA website may be reproduced, distributed and/or used, totally or in part, for non-commercial purposes provided that ECHA is acknowledged as the source: "Source: European Chemicals Agency, http://echa.europa.eu/". Such acknowledgement must be included in each copy of the material. ECHA permits and encourages organisations and individuals to create links to the ECHA website under the following cumulative conditions: Links can only be made to webpages that provide a link to the Legal Notice page. | |
Synthesis routes and methods
Procedure details
Retrosynthesis Analysis
AI-Powered Synthesis Planning: Our tool employs the Template_relevance Pistachio, Template_relevance Bkms_metabolic, Template_relevance Pistachio_ringbreaker, Template_relevance Reaxys, Template_relevance Reaxys_biocatalysis model, leveraging a vast database of chemical reactions to predict feasible synthetic routes.
One-Step Synthesis Focus: Specifically designed for one-step synthesis, it provides concise and direct routes for your target compounds, streamlining the synthesis process.
Accurate Predictions: Utilizing the extensive PISTACHIO, BKMS_METABOLIC, PISTACHIO_RINGBREAKER, REAXYS, REAXYS_BIOCATALYSIS database, our tool offers high-accuracy predictions, reflecting the latest in chemical research and data.
Strategy Settings
Precursor scoring | Relevance Heuristic |
---|---|
Min. plausibility | 0.01 |
Model | Template_relevance |
Template Set | Pistachio/Bkms_metabolic/Pistachio_ringbreaker/Reaxys/Reaxys_biocatalysis |
Top-N result to add to graph | 6 |
Feasible Synthetic Routes
Disclaimer and Information on In-Vitro Research Products
Please be aware that all articles and product information presented on BenchChem are intended solely for informational purposes. The products available for purchase on BenchChem are specifically designed for in-vitro studies, which are conducted outside of living organisms. In-vitro studies, derived from the Latin term "in glass," involve experiments performed in controlled laboratory settings using cells or tissues. It is important to note that these products are not categorized as medicines or drugs, and they have not received approval from the FDA for the prevention, treatment, or cure of any medical condition, ailment, or disease. We must emphasize that any form of bodily introduction of these products into humans or animals is strictly prohibited by law. It is essential to adhere to these guidelines to ensure compliance with legal and ethical standards in research and experimentation.