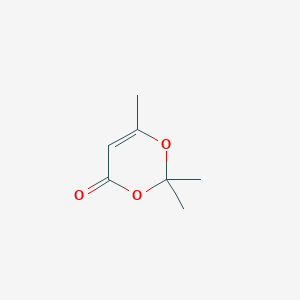
2,2,6-Trimethyl-4H-1,3-dioxin-4-one
Overview
Description
2,2,6-Trimethyl-4H-1,3-dioxin-4-one is used as a building block in organic synthesis and serves as a direct precursor to β-dicarbonyl compounds . It was used in the synthesis of acetylketene by flash pyrolysis .
Synthesis Analysis
The compound is used as a building block in organic synthesis and serves as a direct precursor to β-dicarbonyl compounds . It was used in the synthesis of acetylketene by flash pyrolysis .Molecular Structure Analysis
The molecular structure of 2,2,6-Trimethyl-4H-1,3-dioxin-4-one is represented by the SMILES stringCC1=CC(=O)OC(C)(C)O1
. The compound has a molecular weight of 142.15 . Chemical Reactions Analysis
Flash pyrolysis of 2,2,6-trimethyl-4H-1,3-dioxin-4-one generates acetylketene . It is a diketene-acetone adduct . The compound was used for acetoacetylation of aliphatic and aromatic alcohols, amines, and thiols .Physical And Chemical Properties Analysis
The compound is a liquid with a refractive index of 1.460 (lit.) . It has a boiling point of 275 °C (lit.), 65-67 °C/2 mmHg (lit.) , and a melting point of 12-13 °C (lit.) . It is insoluble in water and has a density of 1.07 g/mL at 25 °C (lit.) .Scientific Research Applications
Organic Synthesis
2,2,6-Trimethyl-4H-1,3-dioxin-4-one: is widely used as a building block in organic synthesis. It serves as a direct precursor to β-dicarbonyl compounds . This compound is instrumental in the synthesis of acetylketene through flash pyrolysis , which is a crucial intermediate for various synthetic pathways.
Pharmaceuticals
In the pharmaceutical industry, this chemical is utilized for the preparation of intermediates such as (S)-1-(3-ethoxy-4-methoxyphenyl)-2-(methanesulfonyl)ethylamine , an intermediate in the synthesis of Aprepitant , a medication used in chemotherapy-induced nausea and vomiting.
Material Science
The compound’s role in material science is primarily as a reactant for synthesizing acetoacetic esters from aliphatic and aromatic alcohols . These esters have applications in polymer chemistry and coatings.
Chemical Research
Researchers employ 2,2,6-Trimethyl-4H-1,3-dioxin-4-one for acetoacetylation of aliphatic and aromatic alcohols, amines, and thiols . This process is vital for modifying chemical structures to enhance or alter their properties for further study.
Industrial Applications
Industrially, 2,2,6-Trimethyl-4H-1,3-dioxin-4-one can be used to create intermediates for coatings, adhesives, and other materials that require specific chemical properties imparted by β-dicarbonyl compounds .
Mechanism of Action
Target of Action
2,2,6-Trimethyl-4H-1,3-dioxin-4-one, also known as a diketene-acetone adduct , is primarily used as a reactant in organic synthesis . It serves as a direct precursor to β-dicarbonyl compounds .
Mode of Action
The compound interacts with its targets through a process known as acetoacetylation . This process involves the addition of an acetoacetyl group to aliphatic and aromatic alcohols, amines, and thiols . The resulting changes include the formation of new compounds such as acetoacetic esters .
Biochemical Pathways
The primary biochemical pathway affected by 2,2,6-Trimethyl-4H-1,3-dioxin-4-one is the synthesis of acetylketene . This occurs through a process known as flash pyrolysis
Pharmacokinetics
It’s known that the compound is insoluble in water , which may affect its absorption, distribution, metabolism, and excretion (ADME) properties
Result of Action
The molecular and cellular effects of 2,2,6-Trimethyl-4H-1,3-dioxin-4-one’s action primarily involve the formation of new compounds through acetoacetylation . For example, it can be used in the preparation of N-alkenyl acetoacetamides .
Action Environment
Environmental factors can influence the action, efficacy, and stability of 2,2,6-Trimethyl-4H-1,3-dioxin-4-one. For instance, the compound is flammable , and exposure to heat can lead to the generation of acetylketene . Therefore, the storage and handling conditions can significantly impact the compound’s action and stability.
Safety and Hazards
Future Directions
The compound has been used in the synthesis of acetylketene by flash pyrolysis and for acetoacetylation of aliphatic and aromatic alcohols, amines, and thiols . It was also used in the preparation of N-alkenyl acetoacetamides . Future research may explore other potential applications of this compound in organic synthesis.
properties
IUPAC Name |
2,2,6-trimethyl-1,3-dioxin-4-one | |
---|---|---|
Source | PubChem | |
URL | https://pubchem.ncbi.nlm.nih.gov | |
Description | Data deposited in or computed by PubChem | |
InChI |
InChI=1S/C7H10O3/c1-5-4-6(8)10-7(2,3)9-5/h4H,1-3H3 | |
Source | PubChem | |
URL | https://pubchem.ncbi.nlm.nih.gov | |
Description | Data deposited in or computed by PubChem | |
InChI Key |
XFRBXZCBOYNMJP-UHFFFAOYSA-N | |
Source | PubChem | |
URL | https://pubchem.ncbi.nlm.nih.gov | |
Description | Data deposited in or computed by PubChem | |
Canonical SMILES |
CC1=CC(=O)OC(O1)(C)C | |
Source | PubChem | |
URL | https://pubchem.ncbi.nlm.nih.gov | |
Description | Data deposited in or computed by PubChem | |
Molecular Formula |
C7H10O3 | |
Source | PubChem | |
URL | https://pubchem.ncbi.nlm.nih.gov | |
Description | Data deposited in or computed by PubChem | |
DSSTOX Substance ID |
DTXSID10202230 | |
Record name | 2,2,6-Trimethyl-4H-1,3-dioxin-4-one | |
Source | EPA DSSTox | |
URL | https://comptox.epa.gov/dashboard/DTXSID10202230 | |
Description | DSSTox provides a high quality public chemistry resource for supporting improved predictive toxicology. | |
Molecular Weight |
142.15 g/mol | |
Source | PubChem | |
URL | https://pubchem.ncbi.nlm.nih.gov | |
Description | Data deposited in or computed by PubChem | |
Physical Description |
Clear dark brown liquid; mp = 12-13 deg C; [Sigma-Aldrich MSDS] | |
Record name | 2,2,6-Trimethyl-4H-1,3-dioxin-4-one | |
Source | Haz-Map, Information on Hazardous Chemicals and Occupational Diseases | |
URL | https://haz-map.com/Agents/19432 | |
Description | Haz-Map® is an occupational health database designed for health and safety professionals and for consumers seeking information about the adverse effects of workplace exposures to chemical and biological agents. | |
Explanation | Copyright (c) 2022 Haz-Map(R). All rights reserved. Unless otherwise indicated, all materials from Haz-Map are copyrighted by Haz-Map(R). No part of these materials, either text or image may be used for any purpose other than for personal use. Therefore, reproduction, modification, storage in a retrieval system or retransmission, in any form or by any means, electronic, mechanical or otherwise, for reasons other than personal use, is strictly prohibited without prior written permission. | |
Product Name |
2,2,6-Trimethyl-4H-1,3-dioxin-4-one | |
CAS RN |
5394-63-8 | |
Record name | 2,2,6-Trimethyl-4H-1,3-dioxin-4-one | |
Source | CAS Common Chemistry | |
URL | https://commonchemistry.cas.org/detail?cas_rn=5394-63-8 | |
Description | CAS Common Chemistry is an open community resource for accessing chemical information. Nearly 500,000 chemical substances from CAS REGISTRY cover areas of community interest, including common and frequently regulated chemicals, and those relevant to high school and undergraduate chemistry classes. This chemical information, curated by our expert scientists, is provided in alignment with our mission as a division of the American Chemical Society. | |
Explanation | The data from CAS Common Chemistry is provided under a CC-BY-NC 4.0 license, unless otherwise stated. | |
Record name | 2,2,6-Trimethyl-4H-1,3-dioxin-4-one | |
Source | ChemIDplus | |
URL | https://pubchem.ncbi.nlm.nih.gov/substance/?source=chemidplus&sourceid=0005394638 | |
Description | ChemIDplus is a free, web search system that provides access to the structure and nomenclature authority files used for the identification of chemical substances cited in National Library of Medicine (NLM) databases, including the TOXNET system. | |
Record name | 5394-63-8 | |
Source | DTP/NCI | |
URL | https://dtp.cancer.gov/dtpstandard/servlet/dwindex?searchtype=NSC&outputformat=html&searchlist=2391 | |
Description | The NCI Development Therapeutics Program (DTP) provides services and resources to the academic and private-sector research communities worldwide to facilitate the discovery and development of new cancer therapeutic agents. | |
Explanation | Unless otherwise indicated, all text within NCI products is free of copyright and may be reused without our permission. Credit the National Cancer Institute as the source. | |
Record name | 2,2,6-Trimethyl-4H-1,3-dioxin-4-one | |
Source | EPA DSSTox | |
URL | https://comptox.epa.gov/dashboard/DTXSID10202230 | |
Description | DSSTox provides a high quality public chemistry resource for supporting improved predictive toxicology. | |
Record name | 2,2,6-trimethyl-4H-1,3-dioxin-4-one | |
Source | European Chemicals Agency (ECHA) | |
URL | https://echa.europa.eu/substance-information/-/substanceinfo/100.024.003 | |
Description | The European Chemicals Agency (ECHA) is an agency of the European Union which is the driving force among regulatory authorities in implementing the EU's groundbreaking chemicals legislation for the benefit of human health and the environment as well as for innovation and competitiveness. | |
Explanation | Use of the information, documents and data from the ECHA website is subject to the terms and conditions of this Legal Notice, and subject to other binding limitations provided for under applicable law, the information, documents and data made available on the ECHA website may be reproduced, distributed and/or used, totally or in part, for non-commercial purposes provided that ECHA is acknowledged as the source: "Source: European Chemicals Agency, http://echa.europa.eu/". Such acknowledgement must be included in each copy of the material. ECHA permits and encourages organisations and individuals to create links to the ECHA website under the following cumulative conditions: Links can only be made to webpages that provide a link to the Legal Notice page. | |
Synthesis routes and methods I
Procedure details
Synthesis routes and methods II
Procedure details
Retrosynthesis Analysis
AI-Powered Synthesis Planning: Our tool employs the Template_relevance Pistachio, Template_relevance Bkms_metabolic, Template_relevance Pistachio_ringbreaker, Template_relevance Reaxys, Template_relevance Reaxys_biocatalysis model, leveraging a vast database of chemical reactions to predict feasible synthetic routes.
One-Step Synthesis Focus: Specifically designed for one-step synthesis, it provides concise and direct routes for your target compounds, streamlining the synthesis process.
Accurate Predictions: Utilizing the extensive PISTACHIO, BKMS_METABOLIC, PISTACHIO_RINGBREAKER, REAXYS, REAXYS_BIOCATALYSIS database, our tool offers high-accuracy predictions, reflecting the latest in chemical research and data.
Strategy Settings
Precursor scoring | Relevance Heuristic |
---|---|
Min. plausibility | 0.01 |
Model | Template_relevance |
Template Set | Pistachio/Bkms_metabolic/Pistachio_ringbreaker/Reaxys/Reaxys_biocatalysis |
Top-N result to add to graph | 6 |
Feasible Synthetic Routes
Q & A
Q1: What is the primary use of 2,2,6-Trimethyl-4H-1,3-dioxin-4-one in organic synthesis?
A1: 2,2,6-Trimethyl-4H-1,3-dioxin-4-one serves as a valuable synthetic equivalent of acetylketene [, ]. This means it readily decomposes upon heating to release acetylketene, a reactive intermediate useful in various organic reactions.
Q2: Can you elaborate on the reactivity of acetylketene generated from 2,2,6-Trimethyl-4H-1,3-dioxin-4-one?
A2: Acetylketene demonstrates high reactivity towards nucleophiles [, , , ]. Studies have shown its reaction with amines to be favored over alcohols, which in turn are more reactive than aldehydes and ketones []. This reactivity pattern is consistent with theoretical predictions based on activation energies and transition state calculations [].
Q3: What specific reactions benefit from using 2,2,6-Trimethyl-4H-1,3-dioxin-4-one as an acetylketene source?
A3: A wide range of reactions utilize 2,2,6-Trimethyl-4H-1,3-dioxin-4-one. Some notable examples include:
- Acetoacetylation: It enables the introduction of acetoacetyl groups into various compounds, including amines [, , ], alcohols [, ], and even polymers like O-(hydroxypropyl)cellulose [].
- Heterocycle Synthesis: It acts as a building block for synthesizing diverse heterocyclic compounds, including pyridines [], dihydropyrimidines [, , ], thiouracils [], and benzo[b][1,4]diazepines [].
- β-Keto Ester and Amide Synthesis: It provides a mild and efficient route to β-keto esters and amides through reaction with alcohols and amines, respectively [].
Q4: Are there any advantages to using 2,2,6-Trimethyl-4H-1,3-dioxin-4-one over diketene directly?
A4: Yes, several advantages make 2,2,6-Trimethyl-4H-1,3-dioxin-4-one preferable:
- Safer Handling: Diketene is a highly reactive and toxic liquid, whereas 2,2,6-Trimethyl-4H-1,3-dioxin-4-one is a safer, easier-to-handle solid [].
- Controlled Release: 2,2,6-Trimethyl-4H-1,3-dioxin-4-one releases acetylketene upon heating, allowing for controlled generation and reaction of this reactive intermediate [].
- Improved Selectivity: The controlled release of acetylketene can lead to improved chemoselectivity in certain reactions [].
Q5: Are there any studies investigating the mechanism of acetylketene generation from 2,2,6-Trimethyl-4H-1,3-dioxin-4-one?
A6: Yes, computational studies have been conducted to understand the thermal decomposition of 2,2,6-Trimethyl-4H-1,3-dioxin-4-one [, ]. These studies suggest that the decomposition could proceed through either a retro-Diels-Alder reaction or a concerted nucleophilic attack mechanism [].
Q6: What spectroscopic techniques are commonly employed to characterize 2,2,6-Trimethyl-4H-1,3-dioxin-4-one and its derivatives?
A6: Commonly used techniques include:
- Nuclear Magnetic Resonance (NMR) Spectroscopy: 1H-NMR and 13C-NMR are essential for structural elucidation, providing information about the types and environments of hydrogen and carbon atoms in the molecule [, ].
- Gas Chromatography (GC): Useful for assessing the purity of 2,2,6-Trimethyl-4H-1,3-dioxin-4-one, particularly regarding acetone contamination [, ].
Q7: Have there been any investigations into modifying the structure of 2,2,6-Trimethyl-4H-1,3-dioxin-4-one to generate other ketenes?
A8: Yes, researchers have explored the functionalization of 2,2,6-Trimethyl-4H-1,3-dioxin-4-one to access substituted acylketenes [, ]. This strategy expands the scope of accessible products by utilizing diverse nucleophiles in reactions with the generated ketenes.
Q8: What are the implications of the findings on acetylketene's reactivity for the design of future reactions?
A9: Understanding the reactivity trends of acetylketene, especially its preference for amines, allows for the strategic planning of multicomponent reactions [, , , ]. This knowledge enables the development of efficient and selective synthetic routes to complex molecules, including those with potential pharmaceutical applications.
Q9: Are there any known environmental concerns regarding 2,2,6-Trimethyl-4H-1,3-dioxin-4-one and its use in synthesis?
A10: While specific information regarding the environmental impact of 2,2,6-Trimethyl-4H-1,3-dioxin-4-one is limited in the provided articles, it is essential to consider waste management and potential ecotoxicological effects when using this compound in synthesis. Exploring alternative solvents, such as water [, ], can contribute to greener synthetic practices.
Disclaimer and Information on In-Vitro Research Products
Please be aware that all articles and product information presented on BenchChem are intended solely for informational purposes. The products available for purchase on BenchChem are specifically designed for in-vitro studies, which are conducted outside of living organisms. In-vitro studies, derived from the Latin term "in glass," involve experiments performed in controlled laboratory settings using cells or tissues. It is important to note that these products are not categorized as medicines or drugs, and they have not received approval from the FDA for the prevention, treatment, or cure of any medical condition, ailment, or disease. We must emphasize that any form of bodily introduction of these products into humans or animals is strictly prohibited by law. It is essential to adhere to these guidelines to ensure compliance with legal and ethical standards in research and experimentation.