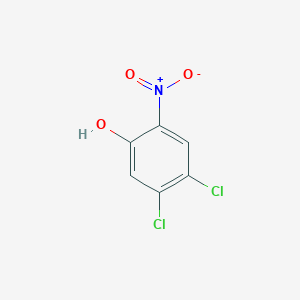
4,5-Dichloro-2-nitrophenol
Overview
Description
4,5-Dichloro-2-nitrophenol is an aromatic compound with the molecular formula C6H3Cl2NO3 It is characterized by the presence of two chlorine atoms and one nitro group attached to a phenol ring
Mechanism of Action
Target of Action
The primary targets of 4,5-Dichloro-2-nitrophenol are G protein alpha subunits (G α) . These proteins play a crucial role in signal transduction from G protein-coupled receptors (GPCRs), which are involved in a wide range of physiological processes.
Mode of Action
This compound interacts with its targets by binding to the G α subunits, thereby potentially affecting the signaling transduction from GPCRs
Biochemical Pathways
The degradation of this compound involves the 1,2,4-benzenetriol (BT) pathway . This pathway is significantly different from the (chloro)hydroquinone pathways reported in other Gram-negative 2C4NP-utilizers . The hnpAB
genes, encoding a two-component FAD-dependent monooxygenase, catalyze the conversion of 2C4NP to BT via chloro-1,4-benzoquinone .
Result of Action
It is known that the compound can inhibit certain reactions, such as the sulfation of p-nitrophenol (p-np) or dopamine . This suggests that this compound may have a significant impact on cellular processes.
Action Environment
Environmental factors can influence the action, efficacy, and stability of this compound. For instance, the compound’s degradation via the BT pathway can be affected by the presence of other substances in the environment
Biochemical Analysis
Cellular Effects
Nitrophenols and their derivatives are known to have toxic effects on cells
Molecular Mechanism
Nitrophenols and their derivatives are known to exert their effects at the molecular level through various mechanisms, including binding interactions with biomolecules, enzyme inhibition or activation, and changes in gene expression .
Dosage Effects in Animal Models
Nitrophenols and their derivatives are known to have toxic or adverse effects at high doses .
Preparation Methods
Synthetic Routes and Reaction Conditions
4,5-Dichloro-2-nitrophenol can be synthesized through the nitration of 4,5-dichlorophenol. The reaction typically involves the use of concentrated nitric acid and sulfuric acid as catalysts. The process is carried out under controlled temperature conditions to ensure the selective nitration of the phenol ring.
Industrial Production Methods
In an industrial setting, the production of this compound involves large-scale nitration reactions. The process includes the careful handling of reagents and the use of advanced equipment to maintain reaction conditions. The product is then purified through crystallization and filtration techniques to achieve the desired purity.
Chemical Reactions Analysis
Types of Reactions
4,5-Dichloro-2-nitrophenol undergoes various chemical reactions, including:
Reduction: The nitro group can be reduced to an amino group using reducing agents such as hydrogen gas in the presence of a catalyst.
Substitution: The chlorine atoms can be substituted with other functional groups through nucleophilic aromatic substitution reactions.
Common Reagents and Conditions
Reduction: Common reagents include hydrogen gas and palladium on carbon as a catalyst.
Substitution: Reagents such as sodium hydroxide or potassium hydroxide can be used for nucleophilic substitution reactions.
Major Products Formed
Reduction: The major product is 4,5-dichloro-2-aminophenol.
Substitution: Depending on the substituent introduced, various derivatives of this compound can be formed.
Scientific Research Applications
4,5-Dichloro-2-nitrophenol has several applications in scientific research:
Chemistry: It is used as an intermediate in the synthesis of more complex organic compounds.
Biology: The compound is studied for its potential effects on biological systems and its role in biochemical pathways.
Medicine: Research is ongoing to explore its potential use in pharmaceuticals.
Industry: It is used in the production of dyes, pesticides, and other industrial chemicals.
Comparison with Similar Compounds
Similar Compounds
- 2-Chloro-4-nitrophenol
- 2-Chloro-5-nitrophenol
- 4-Chloro-2-nitrophenol
- 2,6-Dichloro-4-nitrophenol
Uniqueness
4,5-Dichloro-2-nitrophenol is unique due to the specific positioning of its chlorine and nitro groups, which confer distinct chemical properties and reactivity. This makes it a valuable compound for specific applications where these properties are advantageous.
Properties
IUPAC Name |
4,5-dichloro-2-nitrophenol | |
---|---|---|
Source | PubChem | |
URL | https://pubchem.ncbi.nlm.nih.gov | |
Description | Data deposited in or computed by PubChem | |
InChI |
InChI=1S/C6H3Cl2NO3/c7-3-1-5(9(11)12)6(10)2-4(3)8/h1-2,10H | |
Source | PubChem | |
URL | https://pubchem.ncbi.nlm.nih.gov | |
Description | Data deposited in or computed by PubChem | |
InChI Key |
CEFFUJIHZMPDID-UHFFFAOYSA-N | |
Source | PubChem | |
URL | https://pubchem.ncbi.nlm.nih.gov | |
Description | Data deposited in or computed by PubChem | |
Canonical SMILES |
C1=C(C(=CC(=C1Cl)Cl)O)[N+](=O)[O-] | |
Source | PubChem | |
URL | https://pubchem.ncbi.nlm.nih.gov | |
Description | Data deposited in or computed by PubChem | |
Molecular Formula |
C6H3Cl2NO3 | |
Source | PubChem | |
URL | https://pubchem.ncbi.nlm.nih.gov | |
Description | Data deposited in or computed by PubChem | |
DSSTOX Substance ID |
DTXSID10192485 | |
Record name | 4,5-Dichloro-2-nitrophenol | |
Source | EPA DSSTox | |
URL | https://comptox.epa.gov/dashboard/DTXSID10192485 | |
Description | DSSTox provides a high quality public chemistry resource for supporting improved predictive toxicology. | |
Molecular Weight |
208.00 g/mol | |
Source | PubChem | |
URL | https://pubchem.ncbi.nlm.nih.gov | |
Description | Data deposited in or computed by PubChem | |
CAS No. |
39224-65-2 | |
Record name | 4,5-Dichloro-2-nitrophenol | |
Source | ChemIDplus | |
URL | https://pubchem.ncbi.nlm.nih.gov/substance/?source=chemidplus&sourceid=0039224652 | |
Description | ChemIDplus is a free, web search system that provides access to the structure and nomenclature authority files used for the identification of chemical substances cited in National Library of Medicine (NLM) databases, including the TOXNET system. | |
Record name | 4,5-Dichloro-2-nitrophenol | |
Source | EPA DSSTox | |
URL | https://comptox.epa.gov/dashboard/DTXSID10192485 | |
Description | DSSTox provides a high quality public chemistry resource for supporting improved predictive toxicology. | |
Record name | 4,5-Dichloro-2-nitrophenol | |
Source | European Chemicals Agency (ECHA) | |
URL | https://echa.europa.eu/information-on-chemicals | |
Description | The European Chemicals Agency (ECHA) is an agency of the European Union which is the driving force among regulatory authorities in implementing the EU's groundbreaking chemicals legislation for the benefit of human health and the environment as well as for innovation and competitiveness. | |
Explanation | Use of the information, documents and data from the ECHA website is subject to the terms and conditions of this Legal Notice, and subject to other binding limitations provided for under applicable law, the information, documents and data made available on the ECHA website may be reproduced, distributed and/or used, totally or in part, for non-commercial purposes provided that ECHA is acknowledged as the source: "Source: European Chemicals Agency, http://echa.europa.eu/". Such acknowledgement must be included in each copy of the material. ECHA permits and encourages organisations and individuals to create links to the ECHA website under the following cumulative conditions: Links can only be made to webpages that provide a link to the Legal Notice page. | |
Synthesis routes and methods I
Procedure details
Synthesis routes and methods II
Procedure details
Retrosynthesis Analysis
AI-Powered Synthesis Planning: Our tool employs the Template_relevance Pistachio, Template_relevance Bkms_metabolic, Template_relevance Pistachio_ringbreaker, Template_relevance Reaxys, Template_relevance Reaxys_biocatalysis model, leveraging a vast database of chemical reactions to predict feasible synthetic routes.
One-Step Synthesis Focus: Specifically designed for one-step synthesis, it provides concise and direct routes for your target compounds, streamlining the synthesis process.
Accurate Predictions: Utilizing the extensive PISTACHIO, BKMS_METABOLIC, PISTACHIO_RINGBREAKER, REAXYS, REAXYS_BIOCATALYSIS database, our tool offers high-accuracy predictions, reflecting the latest in chemical research and data.
Strategy Settings
Precursor scoring | Relevance Heuristic |
---|---|
Min. plausibility | 0.01 |
Model | Template_relevance |
Template Set | Pistachio/Bkms_metabolic/Pistachio_ringbreaker/Reaxys/Reaxys_biocatalysis |
Top-N result to add to graph | 6 |
Feasible Synthetic Routes
Disclaimer and Information on In-Vitro Research Products
Please be aware that all articles and product information presented on BenchChem are intended solely for informational purposes. The products available for purchase on BenchChem are specifically designed for in-vitro studies, which are conducted outside of living organisms. In-vitro studies, derived from the Latin term "in glass," involve experiments performed in controlled laboratory settings using cells or tissues. It is important to note that these products are not categorized as medicines or drugs, and they have not received approval from the FDA for the prevention, treatment, or cure of any medical condition, ailment, or disease. We must emphasize that any form of bodily introduction of these products into humans or animals is strictly prohibited by law. It is essential to adhere to these guidelines to ensure compliance with legal and ethical standards in research and experimentation.