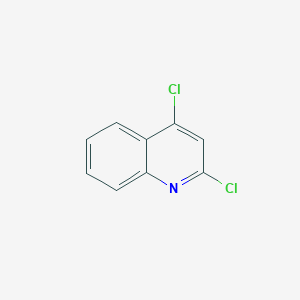
2,4-Dichloroquinoline
Overview
Description
2,4-Dichloroquinoline, with the chemical formula C9H5Cl2N, is a yellow crystalline solid characterized by two chlorine atoms attached to the quinoline ring. This compound is known for its applications in various fields, including pharmaceuticals, agrochemicals, and dyes. It exhibits antimicrobial and antimalarial properties, making it valuable in drug development. Additionally, it is used as a fluorescent probe in biological research and as a ligand in coordination chemistry .
Mechanism of Action
Target of Action
The primary target of 2,4-Dichloroquinoline and its derivatives is the PI3K enzyme . This enzyme plays a crucial role in cellular functions such as cell growth, proliferation, differentiation, motility, survival, and intracellular trafficking, which makes it a significant target in cancer research .
Mode of Action
This compound interacts with its target, the PI3K enzyme, leading to its inhibition . The inhibition of this enzyme disrupts the normal cellular functions it regulates, thereby exerting its cytotoxic effects .
Biochemical Pathways
These effects can include alterations in cell growth and proliferation, potentially leading to cell death .
Result of Action
The molecular and cellular effects of this compound’s action primarily involve cytotoxic activity. The compound has been shown to exhibit significant anticancer activity, with certain derivatives showing better or comparable activity to 2′, 7′-dichlorofluorescein, a reference drug .
Biochemical Analysis
Biochemical Properties
The biochemical properties of 2,4-Dichloroquinoline are not fully understood. Quinoline derivatives have been known to interact with various enzymes, proteins, and other biomolecules. For instance, some quinoline derivatives have been found to inhibit tRNA synthetase, showing antibacterial activity against gram-positive bacteria .
Cellular Effects
A derivative of quinoline showed significant larvicidal and pupicidal properties against a malarial and a dengue vector in a study . This suggests that this compound might also have potential effects on cellular processes.
Molecular Mechanism
Quinoline derivatives are known to exert their effects at the molecular level through various mechanisms, including binding interactions with biomolecules, enzyme inhibition or activation, and changes in gene expression .
Preparation Methods
Synthetic Routes and Reaction Conditions: 2,4-Dichloroquinoline can be synthesized through several methods. One common method involves the reaction of 4-chloropyridine with active thionyl chloride, followed by a cyclization reaction to obtain the target product . Another method involves the condensation of 3-chloroaniline with ethoxy diethyl methylene malonate, followed by cyclization, hydrolysis, decarboxylation, and chlorination .
Industrial Production Methods: Industrial production of this compound often employs microwave-assisted synthesis, which is more efficient and environmentally friendly compared to traditional methods. This technique reduces the usage of harmful chemicals and solvents, making it suitable for large-scale production .
Chemical Reactions Analysis
Types of Reactions: 2,4-Dichloroquinoline undergoes various chemical reactions, including:
Nucleophilic Substitution: The chlorine atoms in the quinoline ring can be replaced by nucleophiles such as amines, thiols, and phenols.
Oxidation and Reduction: It can participate in oxidation and reduction reactions, although these are less common compared to substitution reactions.
Common Reagents and Conditions:
Nucleophilic Substitution: Reagents such as potassium carbonate and cesium carbonate are commonly used under solvent-free conditions or in the presence of dimethylformamide (DMF) at elevated temperatures.
Oxidation and Reduction: Specific oxidizing or reducing agents are used depending on the desired transformation.
Major Products Formed:
Substitution Reactions: Products such as 2-aminoquinoline derivatives and 2-thioquinoline derivatives are commonly formed.
Scientific Research Applications
2,4-Dichloroquinoline has a wide range of scientific research applications:
Comparison with Similar Compounds
4,7-Dichloroquinoline: This compound is also used as an intermediate in the synthesis of antimalarial drugs such as chloroquine and hydroxychloroquine.
2-Chloroquinoline: Another similar compound used in the synthesis of various quinoline derivatives.
Uniqueness: 2,4-Dichloroquinoline is unique due to its specific substitution pattern, which imparts distinct chemical properties and reactivity. Its dual chlorine substitution makes it particularly suitable for nucleophilic substitution reactions, allowing for the synthesis of a wide range of derivatives .
Properties
IUPAC Name |
2,4-dichloroquinoline | |
---|---|---|
Source | PubChem | |
URL | https://pubchem.ncbi.nlm.nih.gov | |
Description | Data deposited in or computed by PubChem | |
InChI |
InChI=1S/C9H5Cl2N/c10-7-5-9(11)12-8-4-2-1-3-6(7)8/h1-5H | |
Source | PubChem | |
URL | https://pubchem.ncbi.nlm.nih.gov | |
Description | Data deposited in or computed by PubChem | |
InChI Key |
QNBJYUUUYZVIJP-UHFFFAOYSA-N | |
Source | PubChem | |
URL | https://pubchem.ncbi.nlm.nih.gov | |
Description | Data deposited in or computed by PubChem | |
Canonical SMILES |
C1=CC=C2C(=C1)C(=CC(=N2)Cl)Cl | |
Source | PubChem | |
URL | https://pubchem.ncbi.nlm.nih.gov | |
Description | Data deposited in or computed by PubChem | |
Molecular Formula |
C9H5Cl2N | |
Source | PubChem | |
URL | https://pubchem.ncbi.nlm.nih.gov | |
Description | Data deposited in or computed by PubChem | |
DSSTOX Substance ID |
DTXSID60345843 | |
Record name | 2,4-Dichloroquinoline | |
Source | EPA DSSTox | |
URL | https://comptox.epa.gov/dashboard/DTXSID60345843 | |
Description | DSSTox provides a high quality public chemistry resource for supporting improved predictive toxicology. | |
Molecular Weight |
198.05 g/mol | |
Source | PubChem | |
URL | https://pubchem.ncbi.nlm.nih.gov | |
Description | Data deposited in or computed by PubChem | |
CAS No. |
703-61-7 | |
Record name | 2,4-Dichloroquinoline | |
Source | EPA DSSTox | |
URL | https://comptox.epa.gov/dashboard/DTXSID60345843 | |
Description | DSSTox provides a high quality public chemistry resource for supporting improved predictive toxicology. | |
Record name | 2,4-Dichloroquinoline | |
Source | European Chemicals Agency (ECHA) | |
URL | https://echa.europa.eu/information-on-chemicals | |
Description | The European Chemicals Agency (ECHA) is an agency of the European Union which is the driving force among regulatory authorities in implementing the EU's groundbreaking chemicals legislation for the benefit of human health and the environment as well as for innovation and competitiveness. | |
Explanation | Use of the information, documents and data from the ECHA website is subject to the terms and conditions of this Legal Notice, and subject to other binding limitations provided for under applicable law, the information, documents and data made available on the ECHA website may be reproduced, distributed and/or used, totally or in part, for non-commercial purposes provided that ECHA is acknowledged as the source: "Source: European Chemicals Agency, http://echa.europa.eu/". Such acknowledgement must be included in each copy of the material. ECHA permits and encourages organisations and individuals to create links to the ECHA website under the following cumulative conditions: Links can only be made to webpages that provide a link to the Legal Notice page. | |
Retrosynthesis Analysis
AI-Powered Synthesis Planning: Our tool employs the Template_relevance Pistachio, Template_relevance Bkms_metabolic, Template_relevance Pistachio_ringbreaker, Template_relevance Reaxys, Template_relevance Reaxys_biocatalysis model, leveraging a vast database of chemical reactions to predict feasible synthetic routes.
One-Step Synthesis Focus: Specifically designed for one-step synthesis, it provides concise and direct routes for your target compounds, streamlining the synthesis process.
Accurate Predictions: Utilizing the extensive PISTACHIO, BKMS_METABOLIC, PISTACHIO_RINGBREAKER, REAXYS, REAXYS_BIOCATALYSIS database, our tool offers high-accuracy predictions, reflecting the latest in chemical research and data.
Strategy Settings
Precursor scoring | Relevance Heuristic |
---|---|
Min. plausibility | 0.01 |
Model | Template_relevance |
Template Set | Pistachio/Bkms_metabolic/Pistachio_ringbreaker/Reaxys/Reaxys_biocatalysis |
Top-N result to add to graph | 6 |
Feasible Synthetic Routes
A: A one-pot method involves the condensation of an appropriate primary aromatic amine with malonic acid in the presence of excess phosphorus oxychloride. This method has been shown to produce 2,4-dichloroquinoline and related derivatives in good yields. [, ]
A: Yes, this compound can be synthesized using diphosgene in acetonitrile by reacting it with 2-ethynylaniline and heating at 130°C or 150°C for 12 hours. [, ]
A: The molecular formula of this compound is C9H5Cl2N, and its molecular weight is 198.05 g/mol. []
A: this compound and its derivatives can be characterized using various spectroscopic techniques, including 1H NMR, 13C NMR, IR, EI-MS, and ESI-MS spectroscopy. [, ]
A: The crystal structure of this compound reveals two crystallographically independent molecules in the asymmetric unit. Both molecules exhibit a planar quinoline ring system. []
A: The reaction of this compound with sodium azide depends on the solvent and reaction conditions. In DMF, it leads to regioselective formation of either 4-azido-2-chloroquinolines or 5-azido-tetrazolo[1,5-a]quinolines depending on the amount of sodium azide and the presence of catalysts. [, ]
A: Yes, both regioselective (using solid sodium alkoxide in toluene) and standard (using alcoholic alkoxide solutions) alkoxydehalogenation reactions can be carried out on 2,4-dichloroquinolines. The regioselectivity and product distribution can be analyzed using NMR spectroscopy. [, ]
A: Yes, this compound serves as a versatile precursor for various fused quinoline heterocycles. For instance, it can be used to synthesize pyrroloquinolines, dibenzo[b,g][1,8]naphthyridin-5-ones, and thieno[3,2-c]quinolines. [, , ]
A: The regioselectivity of reactions between this compound and organozinc reagents can be controlled by using different catalysts and additives. For example, palladium complexes promote α-selective coupling, while LiCl favors γ-selective coupling. [, ]
A: Yes, this compound and its derivatives can react with alkylene diols, such as ethylene glycol, propylene glycol, and butylene glycol, to form polyethers. []
Disclaimer and Information on In-Vitro Research Products
Please be aware that all articles and product information presented on BenchChem are intended solely for informational purposes. The products available for purchase on BenchChem are specifically designed for in-vitro studies, which are conducted outside of living organisms. In-vitro studies, derived from the Latin term "in glass," involve experiments performed in controlled laboratory settings using cells or tissues. It is important to note that these products are not categorized as medicines or drugs, and they have not received approval from the FDA for the prevention, treatment, or cure of any medical condition, ailment, or disease. We must emphasize that any form of bodily introduction of these products into humans or animals is strictly prohibited by law. It is essential to adhere to these guidelines to ensure compliance with legal and ethical standards in research and experimentation.