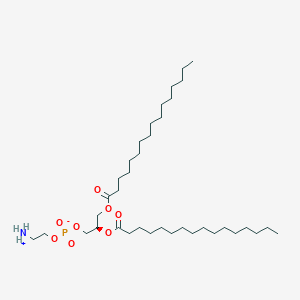
1,2-Dipalmitoyl-sn-glycero-3-phosphoethanolamine
Overview
Description
Mechanism of Action
Target of Action
1,2-Dipalmitoyl-sn-glycero-3-phosphoethanolamine (DPPE), also known as 1,2-dihexadecanoyl-sn-glycero-3-phosphoethanolamine, is a phospholipid that is a major component of the lipid bilayer that surrounds cells . It provides stability to the membrane .
Mode of Action
DPPE interacts with other components of the cell membrane, such as cholesterol . Studies have shown that cholesterol induces condensation of DPPE monolayers in the presence of Ca2+ ions . This interaction can influence the physical properties of the membrane, including its fluidity and permeability.
Biochemical Pathways
DPPE is involved in the phospholipid translocation process across the plasma membrane . ATP11A and ATP11C, members of the P4-ATPase family, translocate phosphatidylserine (PS) and phosphatidylethanolamine from the exoplasmic to the cytoplasmic leaflets at the plasma membrane . This translocation is crucial for maintaining the asymmetric distribution of phospholipids in the cell membrane, which is important for various cellular functions.
Result of Action
The primary result of DPPE’s action is the maintenance of membrane stability and function . By contributing to the lipid bilayer structure of cell membranes, DPPE plays a crucial role in maintaining the integrity of cells and their compartments. It also influences membrane dynamics, which are essential for processes such as vesicle formation, fusion, and fission.
Action Environment
The action of DPPE can be influenced by various environmental factors. For instance, the presence of other lipids, such as cholesterol, can affect the behavior of DPPE in the membrane . Additionally, factors such as pH, temperature, and the presence of ions (like Ca2+) can also influence the properties of the membrane and thus the action of DPPE .
Biochemical Analysis
Biochemical Properties
1,2-Dipalmitoyl-sn-glycero-3-phosphoethanolamine plays a crucial role in biochemical reactions. It interacts with various enzymes, proteins, and other biomolecules. It is a phospholipid, a lipid component in the cell membrane
Cellular Effects
The effects of this compound on various types of cells and cellular processes are significant. It influences cell function, including impact on cell signaling pathways, gene expression, and cellular metabolism .
Molecular Mechanism
The molecular mechanism of action of this compound is intricate. It exerts its effects at the molecular level, including binding interactions with biomolecules, enzyme inhibition or activation, and changes in gene expression .
Temporal Effects in Laboratory Settings
In laboratory settings, the effects of this compound change over time. This includes information on the product’s stability, degradation, and any long-term effects on cellular function observed in in vitro or in vivo studies .
Dosage Effects in Animal Models
The effects of this compound vary with different dosages in animal models. This includes any threshold effects observed in these studies, as well as any toxic or adverse effects at high doses .
Metabolic Pathways
This compound is involved in several metabolic pathways, interacting with various enzymes or cofactors. This also includes any effects on metabolic flux or metabolite levels .
Transport and Distribution
This compound is transported and distributed within cells and tissues. This includes any transporters or binding proteins that it interacts with, as well as any effects on its localization or accumulation .
Subcellular Localization
The subcellular localization of this compound and any effects on its activity or function are complex. This includes any targeting signals or post-translational modifications that direct it to specific compartments or organelles .
Preparation Methods
Synthetic Routes and Reaction Conditions: 1,2-Dipalmitoyl-sn-glycero-3-phosphoethanolamine can be synthesized through the esterification of glycerol with palmitic acid, followed by the phosphorylation of the resulting diacylglycerol with phosphoethanolamine . The reaction typically involves the use of catalysts and specific reaction conditions to ensure high yield and purity .
Industrial Production Methods: In industrial settings, the production of this compound often involves large-scale esterification and phosphorylation processes. These methods are optimized for efficiency and cost-effectiveness, utilizing advanced techniques such as continuous flow reactors and high-pressure conditions .
Chemical Reactions Analysis
Types of Reactions: 1,2-Dipalmitoyl-sn-glycero-3-phosphoethanolamine undergoes various chemical reactions, including oxidation, reduction, and substitution .
Common Reagents and Conditions:
Reduction: Can be achieved using reducing agents like sodium borohydride or lithium aluminum hydride.
Substitution: Often involves nucleophilic substitution reactions with reagents such as alkyl halides or acyl chlorides.
Major Products: The major products formed from these reactions include modified phospholipids with altered fatty acid chains or head groups, which can have different biological properties and applications .
Scientific Research Applications
1,2-Dipalmitoyl-sn-glycero-3-phosphoethanolamine is widely used in scientific research due to its role in cell membrane structure and function . Some of its applications include:
Comparison with Similar Compounds
1,2-Dipalmitoyl-sn-glycero-3-phosphocholine: Another phospholipid with similar structural properties but different head group, leading to distinct biological functions.
1,2-Distearoyl-sn-glycero-3-phosphoethanolamine: Similar to 1,2-Dipalmitoyl-sn-glycero-3-phosphoethanolamine but with stearic acid instead of palmitic acid, resulting in different physical properties.
Uniqueness: this compound is unique due to its specific fatty acid composition and head group, which confer distinct biophysical properties and biological functions . Its ability to form stable lipid bilayers makes it particularly valuable in research and industrial applications .
Properties
CAS No. |
923-61-5 |
---|---|
Molecular Formula |
C37H74NO8P |
Molecular Weight |
692.0 g/mol |
IUPAC Name |
2-azaniumylethyl [(2R)-2,3-di(hexadecanoyloxy)propyl] phosphate |
InChI |
InChI=1S/C37H74NO8P/c1-3-5-7-9-11-13-15-17-19-21-23-25-27-29-36(39)43-33-35(34-45-47(41,42)44-32-31-38)46-37(40)30-28-26-24-22-20-18-16-14-12-10-8-6-4-2/h35H,3-34,38H2,1-2H3,(H,41,42)/t35-/m1/s1 |
InChI Key |
SLKDGVPOSSLUAI-PGUFJCEWSA-N |
SMILES |
CCCCCCCCCCCCCCCC(=O)OCC(COP(=O)([O-])OCC[NH3+])OC(=O)CCCCCCCCCCCCCCC |
Isomeric SMILES |
CCCCCCCCCCCCCCCC(=O)OC[C@H](COP(=O)([O-])OCC[NH3+])OC(=O)CCCCCCCCCCCCCCC |
Canonical SMILES |
CCCCCCCCCCCCCCCC(=O)OCC(COP(=O)([O-])OCC[NH3+])OC(=O)CCCCCCCCCCCCCCC |
Appearance |
White to Off-White Solid |
melting_point |
>134°C (dec.) |
Key on ui other cas no. |
923-61-5 |
physical_description |
Solid |
Purity |
98% |
Synonyms |
1,2-dihexadecyl-sn-glycero-3-phosphoethanolamine 1,2-dipalmitoyl-3-phosphatidylethanolamine 1,2-dipalmitoyl-3-phosphatidylethanolamine, (+-)-isomer 1,2-dipalmitoyl-3-phosphatidylethanolamine, (R)-isomer 1,2-dipalmitoyl-3-phosphatidylethanolamine, ion(1-) 1,2-dipalmitoyl-rac-glycerophosphoethanolamine 1,2-dipalmitoyl-sn-glycero-3-phosphoethanolamine DHPE dipalmitoyl cephalin dipalmitoyl phosphatidylethanolamine phosphatidylethanolamine dipalmitoate |
Origin of Product |
United States |
Retrosynthesis Analysis
AI-Powered Synthesis Planning: Our tool employs the Template_relevance Pistachio, Template_relevance Bkms_metabolic, Template_relevance Pistachio_ringbreaker, Template_relevance Reaxys, Template_relevance Reaxys_biocatalysis model, leveraging a vast database of chemical reactions to predict feasible synthetic routes.
One-Step Synthesis Focus: Specifically designed for one-step synthesis, it provides concise and direct routes for your target compounds, streamlining the synthesis process.
Accurate Predictions: Utilizing the extensive PISTACHIO, BKMS_METABOLIC, PISTACHIO_RINGBREAKER, REAXYS, REAXYS_BIOCATALYSIS database, our tool offers high-accuracy predictions, reflecting the latest in chemical research and data.
Strategy Settings
Precursor scoring | Relevance Heuristic |
---|---|
Min. plausibility | 0.01 |
Model | Template_relevance |
Template Set | Pistachio/Bkms_metabolic/Pistachio_ringbreaker/Reaxys/Reaxys_biocatalysis |
Top-N result to add to graph | 6 |
Feasible Synthetic Routes
Q1: What is the molecular formula and weight of DPPE?
A1: The molecular formula of DPPE is C35H70NO8P, and its molecular weight is 635.9 g/mol.
Q2: Are there any specific spectroscopic data available for DPPE characterization?
A2: Yes, various spectroscopic techniques are used to characterize DPPE, including:
- Nuclear Magnetic Resonance (NMR) Spectroscopy: 1H, 13C, and 31P NMR can provide detailed information about the structure and dynamics of DPPE in various environments. [, , ]
- Fourier Transform Infrared (FTIR) Spectroscopy: FTIR can identify functional groups and characterize the interactions between DPPE and other molecules. [, , ]
- Mass Spectrometry (MS): MS techniques, such as matrix-enhanced secondary ion mass spectrometry (ME SIMS), are useful for identifying and quantifying DPPE in complex mixtures. []
Q3: How does DPPE behave in different environments?
A3: DPPE is a major component of cell membranes and plays a crucial role in membrane structure and function. In aqueous solutions, DPPE forms bilayer structures, mimicking biological membranes, making it a valuable tool in model membrane studies. [, , , , ]
Q4: What factors can impact DPPE stability?
A4: DPPE stability can be affected by:
- Temperature: DPPE undergoes phase transitions depending on temperature, influencing its organization and interactions within membranes. [, , , ]
- pH: The presence of charged groups in DPPE makes its properties sensitive to pH variations. [, ]
- Presence of other molecules: DPPE interacts with various molecules, such as cholesterol, affecting its packing and dynamics within the membrane. [, , , ]
Q5: What are some of the applications of DPPE?
A5: DPPE has diverse applications:
- Model Membranes: DPPE forms stable bilayers, making it a key component in artificial membrane systems used to study membrane properties, protein-lipid interactions, and drug delivery. [, , , , , ]
- Drug Delivery: DPPE is incorporated into liposomes and other nanocarriers for drug delivery applications. Its ability to interact with cell membranes and its biocompatibility makes it a suitable material for targeted drug delivery. [, , , , , ]
- Biosensors: DPPE can be used in biosensor development due to its ability to immobilize enzymes and its sensitivity to changes in the surrounding environment. [, ]
Q6: How is DPPE utilized in drug delivery and targeting?
A6: DPPE is frequently incorporated into liposomes and nanoparticles as a key component for drug delivery systems:
- Liposome Formation: DPPE, with its hydrophobic tail and hydrophilic head group, contributes to forming stable liposomes capable of encapsulating both hydrophilic and hydrophobic drugs. [, , , , , ]
- Targeted Delivery: DPPE can be conjugated with targeting ligands, such as antibodies or peptides, allowing for specific delivery of therapeutic agents to desired cells or tissues. [, ]
- Controlled Release: The incorporation of DPPE in drug delivery systems can influence the release rate of encapsulated drugs, providing sustained release profiles. [, , , ]
Q7: How is computational chemistry used to study DPPE?
A7: Computational techniques provide insights into the behavior of DPPE at the molecular level.
- Molecular Dynamics (MD) Simulations: MD simulations are used to study the structural and dynamic properties of DPPE-containing membranes, including interactions with other lipids, proteins, and drugs. [, , , ]
Q8: How does DPPE interact with other molecules in biological systems?
A8: DPPE interacts with:
- Cholesterol: Cholesterol influences the packing and fluidity of DPPE-containing membranes, modulating membrane properties. [, ]
- Proteins: DPPE interacts with membrane proteins, influencing their structure, function, and localization. [, ]
- Drugs: The interaction of DPPE with drugs can influence drug partitioning into membranes, affecting drug efficacy and delivery. [, , ]
Disclaimer and Information on In-Vitro Research Products
Please be aware that all articles and product information presented on BenchChem are intended solely for informational purposes. The products available for purchase on BenchChem are specifically designed for in-vitro studies, which are conducted outside of living organisms. In-vitro studies, derived from the Latin term "in glass," involve experiments performed in controlled laboratory settings using cells or tissues. It is important to note that these products are not categorized as medicines or drugs, and they have not received approval from the FDA for the prevention, treatment, or cure of any medical condition, ailment, or disease. We must emphasize that any form of bodily introduction of these products into humans or animals is strictly prohibited by law. It is essential to adhere to these guidelines to ensure compliance with legal and ethical standards in research and experimentation.