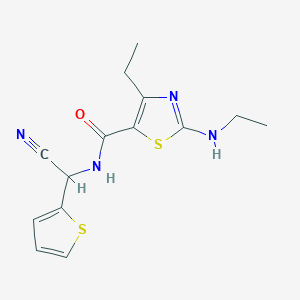
Ethaboxam
Overview
Description
Ethaboxam is a systemic fungicide belonging to the thiazole carboxamide class. It is primarily used to control soil-borne pathogens, particularly those caused by oomycetes such as Phytophthora and Pythium species. This compound is known for its effectiveness in protecting crops like potatoes, grapes, and various vegetables from diseases like late blight and downy mildew .
Mechanism of Action
Target of Action
Ethaboxam is a thiazole carboxamide fungicide that primarily targets various diseases caused by oomycetes . According to the Fungicide Resistance Action Committee (FRAC), this compound belongs to Group 22 fungicides that affect β-tubulin assembly in mitosis . β-tubulin is a protein dimer, which consists of α- and β-subunits and is the main component of microtubule filaments, playing a central role in nuclear division in all eukaryotic cells .
Mode of Action
This compound is believed to have multiple sites of action as it inhibits migration of nuclei from the growing germ tube and mycelia, and appears to inhibit oxygen consumption by mitochondria . This interaction with its targets results in the disruption of the normal cellular processes of the pathogens, thereby inhibiting their growth and proliferation .
Biochemical Pathways
It is known that the compound interferes with the assembly of β-tubulin during mitosis . This disruption of the microtubule dynamics affects the normal cell division process, leading to the death of the pathogenic organisms .
Pharmacokinetics
This compound has a low aqueous solubility and is considered to be volatile . Although it has a low mammalian toxicity, it has a high potential to bioaccumulate . These ADME properties can impact the bioavailability of the compound, influencing its effectiveness as a fungicide.
Result of Action
The molecular and cellular effects of this compound’s action result in the inhibition of mycelial growth of pathogens such as Phytophthora infestans and P capsici . In terms of preventative activity, this compound almost completely suppressed the development of various plant diseases . It is also found to be persistent, maintaining its preventative activity even days after treatment .
Action Environment
Environmental factors can influence the action, efficacy, and stability of this compound. For instance, the compound is toxic to aquatic organisms, and drift and runoff from treated areas may be hazardous to aquatic organisms in water adjacent to treated areas . Therefore, the application of this compound must be managed carefully to minimize environmental impact. Furthermore, the effectiveness of this compound can be influenced by the specific environmental conditions of the area where it is applied, such as soil type and climate .
Biochemical Analysis
Biochemical Properties
Ethaboxam interacts with various biomolecules in its role as a fungicide. It is believed to inhibit mycelial growth and sporulation of certain pathogens .
Cellular Effects
This compound has been shown to have significant effects on cellular processes. It is believed to inhibit migration of nuclei from the cyst to the growing germ tube and mycelia, and appears to inhibit oxygen consumption by mitochondria . This suggests that this compound has a profound impact on cell function, potentially affecting cell signaling pathways, gene expression, and cellular metabolism.
Molecular Mechanism
The molecular mechanism of this compound involves its interaction with β-tubulin . It is part of the benzamide class of fungicides, which inhibit microtub
Preparation Methods
Synthetic Routes and Reaction Conditions: Ethaboxam is synthesized through a multi-step process involving the reaction of thiazole derivatives with various reagents. The key steps include:
Formation of Thiazole Ring: The synthesis begins with the formation of the thiazole ring by reacting α-bromoacetophenone with thiourea.
Introduction of Cyano Group: The cyano group is introduced through a nucleophilic substitution reaction using sodium cyanide.
Ethylation: The thiazole derivative is then ethylated using ethyl iodide under basic conditions.
Amidation: The final step involves the amidation of the thiazole derivative with ethylamine to form this compound
Industrial Production Methods: Industrial production of this compound involves similar synthetic routes but on a larger scale. The process is optimized for high yield and purity, often using continuous flow reactors and advanced purification techniques like crystallization and chromatography .
Chemical Reactions Analysis
Types of Reactions: Ethaboxam undergoes various chemical reactions, including:
Oxidation: this compound can be oxidized to form sulfoxides and sulfones.
Reduction: Reduction reactions can convert this compound to its corresponding amine derivatives.
Substitution: this compound can undergo nucleophilic substitution reactions, particularly at the cyano group.
Common Reagents and Conditions:
Oxidation: Common oxidizing agents include hydrogen peroxide and m-chloroperbenzoic acid.
Reduction: Reducing agents like lithium aluminum hydride and sodium borohydride are used.
Substitution: Nucleophiles such as amines and thiols are commonly used in substitution reactions.
Major Products:
Oxidation: Sulfoxides and sulfones.
Reduction: Amine derivatives.
Substitution: Various substituted thiazole derivatives.
Scientific Research Applications
Ethaboxam has a wide range of scientific research applications:
Chemistry: Used as a model compound for studying thiazole chemistry and its derivatives.
Biology: Investigated for its effects on fungal pathogens and its potential use in controlling plant diseases.
Medicine: Explored for its potential antifungal properties in medical applications.
Industry: Widely used in agriculture for protecting crops from soil-borne pathogens .
Comparison with Similar Compounds
- Mandipropamid
- Fluopicolide
- Oxathiapiprolin
- Metalaxyl
- Mefenoxam
Properties
IUPAC Name |
N-[cyano(thiophen-2-yl)methyl]-4-ethyl-2-(ethylamino)-1,3-thiazole-5-carboxamide | |
---|---|---|
Source | PubChem | |
URL | https://pubchem.ncbi.nlm.nih.gov | |
Description | Data deposited in or computed by PubChem | |
InChI |
InChI=1S/C14H16N4OS2/c1-3-9-12(21-14(18-9)16-4-2)13(19)17-10(8-15)11-6-5-7-20-11/h5-7,10H,3-4H2,1-2H3,(H,16,18)(H,17,19) | |
Source | PubChem | |
URL | https://pubchem.ncbi.nlm.nih.gov | |
Description | Data deposited in or computed by PubChem | |
InChI Key |
NQRFDNJEBWAUBL-UHFFFAOYSA-N | |
Source | PubChem | |
URL | https://pubchem.ncbi.nlm.nih.gov | |
Description | Data deposited in or computed by PubChem | |
Canonical SMILES |
CCC1=C(SC(=N1)NCC)C(=O)NC(C#N)C2=CC=CS2 | |
Source | PubChem | |
URL | https://pubchem.ncbi.nlm.nih.gov | |
Description | Data deposited in or computed by PubChem | |
Molecular Formula |
C14H16N4OS2 | |
Source | PubChem | |
URL | https://pubchem.ncbi.nlm.nih.gov | |
Description | Data deposited in or computed by PubChem | |
DSSTOX Substance ID |
DTXSID2057994 | |
Record name | Ethaboxam | |
Source | EPA DSSTox | |
URL | https://comptox.epa.gov/dashboard/DTXSID2057994 | |
Description | DSSTox provides a high quality public chemistry resource for supporting improved predictive toxicology. | |
Molecular Weight |
320.4 g/mol | |
Source | PubChem | |
URL | https://pubchem.ncbi.nlm.nih.gov | |
Description | Data deposited in or computed by PubChem | |
CAS No. |
162650-77-3 | |
Record name | Ethaboxam | |
Source | CAS Common Chemistry | |
URL | https://commonchemistry.cas.org/detail?cas_rn=162650-77-3 | |
Description | CAS Common Chemistry is an open community resource for accessing chemical information. Nearly 500,000 chemical substances from CAS REGISTRY cover areas of community interest, including common and frequently regulated chemicals, and those relevant to high school and undergraduate chemistry classes. This chemical information, curated by our expert scientists, is provided in alignment with our mission as a division of the American Chemical Society. | |
Explanation | The data from CAS Common Chemistry is provided under a CC-BY-NC 4.0 license, unless otherwise stated. | |
Record name | Ethaboxam [ISO] | |
Source | ChemIDplus | |
URL | https://pubchem.ncbi.nlm.nih.gov/substance/?source=chemidplus&sourceid=0162650773 | |
Description | ChemIDplus is a free, web search system that provides access to the structure and nomenclature authority files used for the identification of chemical substances cited in National Library of Medicine (NLM) databases, including the TOXNET system. | |
Record name | Ethaboxam | |
Source | EPA DSSTox | |
URL | https://comptox.epa.gov/dashboard/DTXSID2057994 | |
Description | DSSTox provides a high quality public chemistry resource for supporting improved predictive toxicology. | |
Record name | ETHABOXAM | |
Source | FDA Global Substance Registration System (GSRS) | |
URL | https://gsrs.ncats.nih.gov/ginas/app/beta/substances/7MP0L17BM8 | |
Description | The FDA Global Substance Registration System (GSRS) enables the efficient and accurate exchange of information on what substances are in regulated products. Instead of relying on names, which vary across regulatory domains, countries, and regions, the GSRS knowledge base makes it possible for substances to be defined by standardized, scientific descriptions. | |
Explanation | Unless otherwise noted, the contents of the FDA website (www.fda.gov), both text and graphics, are not copyrighted. They are in the public domain and may be republished, reprinted and otherwise used freely by anyone without the need to obtain permission from FDA. Credit to the U.S. Food and Drug Administration as the source is appreciated but not required. | |
Synthesis routes and methods
Procedure details
Retrosynthesis Analysis
AI-Powered Synthesis Planning: Our tool employs the Template_relevance Pistachio, Template_relevance Bkms_metabolic, Template_relevance Pistachio_ringbreaker, Template_relevance Reaxys, Template_relevance Reaxys_biocatalysis model, leveraging a vast database of chemical reactions to predict feasible synthetic routes.
One-Step Synthesis Focus: Specifically designed for one-step synthesis, it provides concise and direct routes for your target compounds, streamlining the synthesis process.
Accurate Predictions: Utilizing the extensive PISTACHIO, BKMS_METABOLIC, PISTACHIO_RINGBREAKER, REAXYS, REAXYS_BIOCATALYSIS database, our tool offers high-accuracy predictions, reflecting the latest in chemical research and data.
Strategy Settings
Precursor scoring | Relevance Heuristic |
---|---|
Min. plausibility | 0.01 |
Model | Template_relevance |
Template Set | Pistachio/Bkms_metabolic/Pistachio_ringbreaker/Reaxys/Reaxys_biocatalysis |
Top-N result to add to graph | 6 |
Feasible Synthetic Routes
Q1: What is the mechanism of action of Ethaboxam?
A1: this compound specifically targets and disrupts microtubule organization in Oomycetes. [] Microtubules are essential cellular components involved in various cellular processes, including cell division and growth. By disrupting microtubule integrity, this compound inhibits the growth and development of Oomycetes. []
Q2: Does this compound affect microtubules in other organisms like fungi or mammals?
A2: Studies have shown that this compound specifically targets microtubules in Oomycetes. [] Research using the model fungus Aspergillus nidulans and mammalian mouse cells did not find evidence of microtubule disruption after this compound exposure. []
Q3: What is the chemical structure of this compound?
A3: this compound is a chiral molecule, meaning it exists in two mirror-image forms called enantiomers. Its chemical name is (RS)-N-(α-cyano-2-thenyl)-4-ethyl-2-(ethylamino)-1,3-thiazole-5-carboxamide. []
Q4: Is there a way to separate the enantiomers of this compound?
A4: Yes, researchers have successfully separated the enantiomers of this compound using polysaccharide-derived chiral stationary phases in liquid chromatography. []
Q5: What plant diseases caused by Oomycetes can this compound control?
A5: Research indicates that this compound effectively controls various diseases, including:
- Cucumber downy mildew: Caused by Pseudoperonospora cubensis. [, , , ]
- Potato late blight: Caused by Phytophthora infestans. [, , , , , ]
- Pepper Phytophthora blight: Caused by Phytophthora capsici. [, , ]
- Chinese cabbage clubroot: Caused by Plasmodiophora brassicae. [, , ]
- Soybean damping off and root rot: Caused by various Phytophthora, Phytopythium, and Pythium species. [, , ]
- Chickpea seed rot and damping off: Caused by Pythium ultimum. []
Q6: How is this compound typically applied to control these diseases?
A6: this compound is often used as a seed treatment, [, , , , ] but foliar applications have also proven effective. [, , ] The specific application method and rate may vary depending on the crop, disease, and local regulations.
Q7: Is this compound effective as a seed treatment for managing Phytophthora root rot in soybean?
A7: Field experiments in Illinois and Iowa demonstrated that this compound seed treatments increased soybean stands across various locations and years. [] The efficacy was more pronounced in locations inoculated with Phytophthora spp., with significant yield increases observed. []
Q8: Can this compound be used for the control of ivy stem rot?
A8: Yes, research has shown that this compound, particularly in combination with famoxadone, effectively controls ivy stem rot caused by Phytophthora cinnamomi. []
Q9: Have any instances of this compound resistance been reported?
A9: While this compound resistance is relatively uncommon, there have been reports of reduced sensitivity in some Pythium species. [, ] In Montana, a Pythium sylvaticum isolate showed reduced sensitivity to this compound. []
Q10: Are there naturally occurring instances of this compound insensitivity in Oomycetes?
A10: Yes, research has identified naturally occurring this compound insensitivity in some Pythium species. This insensitivity is linked to a specific mutation in the β-tubulin gene (C239S) and appears to have evolved without direct this compound selection pressure. []
Q11: Does the C239S mutation also occur in other Oomycetes?
A11: Interestingly, the C239S mutation has been found in other Peronosporalean oomycetes, notably within the genus Peronospora, suggesting a broader evolutionary significance of this mutation. []
Q12: Does the use of this compound impact the sensitivity of Phytophthora infestans to other fungicides?
A13: Research in Korea indicates no cross-resistance between this compound and metalaxyl in Phytophthora infestans isolates. Isolates, regardless of their metalaxyl sensitivity, were inhibited by this compound. []
Q13: What is known about the metabolism of this compound?
A14: Studies using rat liver microsomes show that this compound is primarily metabolized through N-deethylation. [, ] Cytochrome P450 monooxygenase enzymes play a key role in this metabolic process. []
Q14: Are there any microbial species capable of degrading this compound?
A15: Research shows that the soil fungus Cunninghamella elegans can biotransform this compound, producing metabolites like N-deethylated this compound and 4-ethyl-2-(ethylamino)-1,3-thiazole-5-carboxamide. []
Q15: Can the efficacy of this compound be improved through formulation techniques?
A16: Yes, incorporating a non-ionic surfactant, polyoxyethylene cetyl ether (PCE), into this compound formulations, particularly wettable powders, has been shown to enhance its fungicidal activity. [] This improvement is attributed to better persistence and curative efficacy. []
Q16: Are there any synergistic effects when this compound is combined with other fungicides?
A16: Research shows that combining this compound with specific fungicides can lead to synergistic effects, enhancing its overall efficacy. Synergistic combinations have been observed with:
- Pyraclostrobin: This combination shows particular promise for controlling downy mildew and other epidemic diseases. []
- Amisulbrom: This mixture effectively controls downy mildew and epidemic diseases in various crops like vegetables, grapes, lychees, and melons. []
Q17: Are there specific recommendations for fungicide rotations or mixtures with this compound?
A18: Research suggests rotating or mixing this compound with fungicides possessing different modes of action, such as oxathiapiprolin, fluopicolide, and mandipropamid, to prevent resistance development. [] This strategy is particularly crucial for managing diseases like avocado root rot. []
Q18: What is the environmental fate of this compound?
A19: While detailed information on the environmental fate and degradation of this compound is limited in the provided research, studies on microbial degradation and the identification of specific metabolites provide some insights. Further research is necessary to fully understand its long-term environmental impact. []
Q19: Does this compound leave residues in crops?
A20: Studies on Korean cabbage indicate that while this compound residues can be present in outer leaves, they are generally not detected in the inner part of the head. [, ] This finding suggests minimal risk associated with consuming the inner parts of treated cabbages. [, ]
Q20: Can this compound residues in outer cabbage leaves be reduced?
A21: Yes, common processing techniques like water washing, blanching, and heat-cooking effectively reduce this compound residues in outer cabbage leaves, making them safer for consumption. [, ]
Q21: What analytical methods are used to detect and quantify this compound?
A22: Liquid chromatography coupled with tandem mass spectrometry (LC/MS/MS) is widely employed for the determination of this compound residues in soil, crops, and other matrices. [, ] This technique offers high sensitivity and selectivity for accurate quantification. [, ]
Q22: How is this compound extracted from different matrices for analysis?
A23: Common extraction methods involve using solvents like acetonitrile, followed by purification steps such as solid-phase extraction using materials like ODS C18-N and PSA. [] This process ensures the removal of interfering compounds and concentrates the analyte for accurate analysis. []
Disclaimer and Information on In-Vitro Research Products
Please be aware that all articles and product information presented on BenchChem are intended solely for informational purposes. The products available for purchase on BenchChem are specifically designed for in-vitro studies, which are conducted outside of living organisms. In-vitro studies, derived from the Latin term "in glass," involve experiments performed in controlled laboratory settings using cells or tissues. It is important to note that these products are not categorized as medicines or drugs, and they have not received approval from the FDA for the prevention, treatment, or cure of any medical condition, ailment, or disease. We must emphasize that any form of bodily introduction of these products into humans or animals is strictly prohibited by law. It is essential to adhere to these guidelines to ensure compliance with legal and ethical standards in research and experimentation.