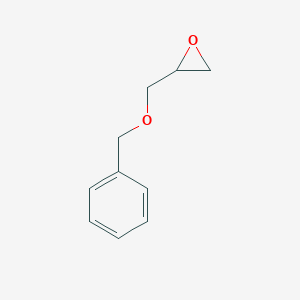
Benzyl glycidyl ether
Overview
Description
Benzyl glycidyl ether is an organic compound that belongs to the class of glycidyl ethers. It is characterized by the presence of a benzyl group attached to a glycidyl ether moiety. This compound is known for its versatility and is widely used as a precursor in the synthesis of various pharmaceuticals and natural products. Optically pure this compound plays a significant role in the production of chiral intermediates, which are essential in the pharmaceutical industry .
Mechanism of Action
Target of Action
Benzyl Glycidyl Ether (BGE) primarily targets the epoxide hydrolase (EH) enzyme . This enzyme is responsible for the hydrolysis of epoxides to their corresponding diols . The interaction of BGE with this enzyme is crucial for its mechanism of action.
Biochemical Pathways
The primary biochemical pathway affected by BGE is the etherification process of glycerol . This process involves various stages of reaction, resulting in the formation of different ether compounds . The etherification of glycerol with 1-butene involves a series of equilibrium reactions to produce mono-ethers, di-ethers, and tri-ethers .
Pharmacokinetics
The biocatalytic resolution of bge by talaromyces flavus has been described, suggesting that microorganisms may play a role in its metabolism .
Result of Action
The result of BGE’s action is the production of optically pure this compound and its derivatives . These compounds play an important role in the synthesis of many drugs and natural products . For example, (S)-BGE is an intermediate of (+)-cryptocarya diacetate, a natural product used in the treatment of headaches, morning sickness, and cancer pulmonary diseases . ®-BGE is an intermediate for synargentoide A, which has cytotoxic, anti-tumor, and anti-leukemic properties .
Action Environment
The action of BGE can be influenced by environmental factors. For instance, the enantioselective hydrolysis of BGE can be optimized under certain fermentation conditions . Additionally, the presence of tetrabutylammonium hydrogen sulphate likely acts as a phase-transfer catalyst to facilitate the reaction . It’s also important to note that BGE should be handled in a well-ventilated place, and contact with skin and eyes should be avoided .
Biochemical Analysis
Biochemical Properties
BGE interacts with a variety of biomolecules, most notably enzymes such as epoxide hydrolase (EH). Talaromyces flavus, a microorganism, contains a constitutive EH that can resolve racemic BGE into its ®-enantiomers . This interaction is crucial for the production of chiral epoxides in the pharmaceutical industry .
Cellular Effects
The cellular effects of BGE are primarily observed in its role as a precursor for optical glycidyl ether, which is used in the synthesis of many drugs and natural products . For instance, (S)-BGE is an intermediate of (+)-cryptocarya diacetate, a natural product used in the treatment of headaches, morning sickness, and cancer pulmonary diseases .
Molecular Mechanism
BGE exerts its effects at the molecular level through its interactions with enzymes like EH. The EH catalyzes the hydrolysis of epoxides to the corresponding diol, a process that is crucial for the production of chiral epoxides .
Temporal Effects in Laboratory Settings
The effects of BGE over time in laboratory settings have been observed in the context of its resolution by Talaromyces flavus. After optimization of the fermentation conditions, the specific EH activity and biomass concentration were improved .
Metabolic Pathways
BGE is involved in the metabolic pathway of the synthesis of many drugs and natural products . It interacts with enzymes like EH in this process .
Preparation Methods
Synthetic Routes and Reaction Conditions: Benzyl glycidyl ether can be synthesized through the reaction of epichlorohydrin with benzyl alcohol in the presence of a phase transfer catalyst such as tetrabutylammonium hydrogen sulfate and aqueous sodium hydroxide. The reaction is typically carried out at low temperatures (0°C) to ensure high yield and selectivity .
Industrial Production Methods: In industrial settings, the production of this compound involves the use of continuous flow reactors to maintain optimal reaction conditions and ensure consistent product quality. The process includes the distillation of the product to achieve high purity levels .
Chemical Reactions Analysis
Types of Reactions: Benzyl glycidyl ether undergoes various chemical reactions, including:
Oxidation: The compound can be oxidized to form corresponding diols.
Reduction: It can be reduced to yield alcohols.
Substitution: this compound can participate in nucleophilic substitution reactions, where the glycidyl group is replaced by other nucleophiles.
Common Reagents and Conditions:
Oxidation: Common oxidizing agents include hydrogen peroxide and peracids.
Reduction: Reducing agents such as lithium aluminum hydride are used.
Substitution: Nucleophiles like amines and thiols are commonly employed.
Major Products Formed:
Oxidation: Diols
Reduction: Alcohols
Substitution: Substituted ethers
Scientific Research Applications
Benzyl glycidyl ether has a wide range of applications in scientific research:
Chemistry: It is used as a building block in the synthesis of complex organic molecules and chiral intermediates.
Biology: The compound is employed in the preparation of biologically active molecules, including enzyme inhibitors and receptor ligands.
Comparison with Similar Compounds
- Methylbenzyl glycidyl ether
- Dimethoxylthis compound
- Styrene oxide
This compound stands out due to its unique combination of reactivity and stability, making it a valuable compound in various scientific and industrial applications.
Properties
IUPAC Name |
2-(phenylmethoxymethyl)oxirane | |
---|---|---|
Source | PubChem | |
URL | https://pubchem.ncbi.nlm.nih.gov | |
Description | Data deposited in or computed by PubChem | |
InChI |
InChI=1S/C10H12O2/c1-2-4-9(5-3-1)6-11-7-10-8-12-10/h1-5,10H,6-8H2 | |
Source | PubChem | |
URL | https://pubchem.ncbi.nlm.nih.gov | |
Description | Data deposited in or computed by PubChem | |
InChI Key |
QNYBOILAKBSWFG-UHFFFAOYSA-N | |
Source | PubChem | |
URL | https://pubchem.ncbi.nlm.nih.gov | |
Description | Data deposited in or computed by PubChem | |
Canonical SMILES |
C1C(O1)COCC2=CC=CC=C2 | |
Source | PubChem | |
URL | https://pubchem.ncbi.nlm.nih.gov | |
Description | Data deposited in or computed by PubChem | |
Molecular Formula |
C10H12O2 | |
Source | PubChem | |
URL | https://pubchem.ncbi.nlm.nih.gov | |
Description | Data deposited in or computed by PubChem | |
DSSTOX Substance ID |
DTXSID001019317 | |
Record name | 2‐[(Benzyloxy)methyl]oxirane | |
Source | EPA DSSTox | |
URL | https://comptox.epa.gov/dashboard/DTXSID001019317 | |
Description | DSSTox provides a high quality public chemistry resource for supporting improved predictive toxicology. | |
Molecular Weight |
164.20 g/mol | |
Source | PubChem | |
URL | https://pubchem.ncbi.nlm.nih.gov | |
Description | Data deposited in or computed by PubChem | |
CAS No. |
2930-05-4 | |
Record name | Benzyl glycidyl ether | |
Source | CAS Common Chemistry | |
URL | https://commonchemistry.cas.org/detail?cas_rn=2930-05-4 | |
Description | CAS Common Chemistry is an open community resource for accessing chemical information. Nearly 500,000 chemical substances from CAS REGISTRY cover areas of community interest, including common and frequently regulated chemicals, and those relevant to high school and undergraduate chemistry classes. This chemical information, curated by our expert scientists, is provided in alignment with our mission as a division of the American Chemical Society. | |
Explanation | The data from CAS Common Chemistry is provided under a CC-BY-NC 4.0 license, unless otherwise stated. | |
Record name | Propane, 1-(benzyloxy)-2,3-epoxy- | |
Source | ChemIDplus | |
URL | https://pubchem.ncbi.nlm.nih.gov/substance/?source=chemidplus&sourceid=0002930054 | |
Description | ChemIDplus is a free, web search system that provides access to the structure and nomenclature authority files used for the identification of chemical substances cited in National Library of Medicine (NLM) databases, including the TOXNET system. | |
Record name | 2‐[(Benzyloxy)methyl]oxirane | |
Source | EPA DSSTox | |
URL | https://comptox.epa.gov/dashboard/DTXSID001019317 | |
Description | DSSTox provides a high quality public chemistry resource for supporting improved predictive toxicology. | |
Synthesis routes and methods I
Procedure details
Synthesis routes and methods II
Procedure details
Synthesis routes and methods III
Procedure details
Retrosynthesis Analysis
AI-Powered Synthesis Planning: Our tool employs the Template_relevance Pistachio, Template_relevance Bkms_metabolic, Template_relevance Pistachio_ringbreaker, Template_relevance Reaxys, Template_relevance Reaxys_biocatalysis model, leveraging a vast database of chemical reactions to predict feasible synthetic routes.
One-Step Synthesis Focus: Specifically designed for one-step synthesis, it provides concise and direct routes for your target compounds, streamlining the synthesis process.
Accurate Predictions: Utilizing the extensive PISTACHIO, BKMS_METABOLIC, PISTACHIO_RINGBREAKER, REAXYS, REAXYS_BIOCATALYSIS database, our tool offers high-accuracy predictions, reflecting the latest in chemical research and data.
Strategy Settings
Precursor scoring | Relevance Heuristic |
---|---|
Min. plausibility | 0.01 |
Model | Template_relevance |
Template Set | Pistachio/Bkms_metabolic/Pistachio_ringbreaker/Reaxys/Reaxys_biocatalysis |
Top-N result to add to graph | 6 |
Feasible Synthetic Routes
Q1: What is the molecular formula and weight of benzyl glycidyl ether?
A1: The molecular formula of this compound is C10H12O2, and its molecular weight is 164.20 g/mol.
Q2: How is this compound typically characterized?
A2: this compound is commonly characterized using techniques like Fourier Transform Infrared Spectroscopy (FTIR) [], Nuclear Magnetic Resonance (NMR) spectroscopy [, ], and Size Exclusion Chromatography (SEC) [] to determine its structure, purity, and molecular weight.
Q3: What are the material compatibility characteristics of BGE?
A3: this compound exhibits compatibility with various materials, including epoxy resins [, ], acrylic acid [, ], and poly(ethylene glycol) []. This compatibility makes it suitable for applications like UV-curable diluents [, ], anti-rust coatings [], and drug delivery systems [].
Q4: How is this compound utilized in organic synthesis?
A4: this compound serves as a valuable building block in organic synthesis, particularly for synthesizing optically active compounds. For instance, it acts as a starting material in the synthesis of 4-deoxy-L-(and D-)hexoses [] and the cytotoxic fatty acid found in the Hericium erinaceum mushroom [].
Q5: What is the role of this compound in polymer synthesis?
A5: this compound acts as a monomer in various polymerization reactions. For example, it is terpolymerized with propylene oxide and CO2 to produce poly(benzyl 1,2-glycerol-co-propylene carbonate)s, showcasing its versatility in developing degradable polymers [].
Q6: Can this compound be polymerized in a controlled manner?
A6: Yes, this compound can undergo controlled ring-opening polymerization (ROP) using catalysts like phosphazene bases. This allows for the synthesis of well-defined protected polyglycidols, enabling the development of end-functionalized polyethers with specific properties [].
Q7: How is this compound used in the development of drug delivery systems?
A7: this compound is incorporated into polymers designed for drug delivery applications. For instance, it plays a crucial role in synthesizing polyethylene glycol-poly(glycerol carbonate) block copolymers that self-assemble into micelles, showing promise as surfactant-free drug carriers [].
Q8: Can this compound be resolved into its enantiomers?
A8: Yes, racemic this compound can be resolved into its enantiomers using biocatalytic approaches. This resolution utilizes enzymes like epoxide hydrolases from various sources, including the yeast Yarrowia lipolytica [] and the bacterium Bacillus alcalophilus [].
Q9: Why is the enantioselective resolution of this compound important?
A9: Enantiopure forms of BGE are crucial in synthesizing chiral pharmaceuticals and other biologically active compounds. The (R)-enantiomer, for example, is a key intermediate in the synthesis of the gastroprokinetic agent mosapride [].
Q10: How do structural modifications to BGE affect its biocatalytic resolution?
A10: Studies on the biocatalytic resolution of BGE derivatives have shown that substituents on the phenyl ring can impact the enantioselectivity of enzymes like Talaromyces flavus epoxide hydrolase. This highlights the importance of structure-activity relationships in optimizing biocatalytic processes [, ].
Q11: How is computational chemistry employed in studying this compound?
A11: Computational methods, such as molecular docking simulations, help researchers understand the interactions between BGE enantiomers and enzymes like epoxide hydrolases. This provides insights into the origin of enantioselectivity and aids in the rational design of more efficient biocatalysts [].
Q12: What is known about the stability of this compound?
A12: While specific stability data may vary depending on the formulation and storage conditions, BGE is generally considered stable under standard laboratory conditions. It is often used as a reactive diluent in epoxy resin systems, suggesting compatibility with curing processes [].
Q13: Are there any safety concerns regarding the use of this compound?
A13: As with any chemical compound, proper handling and safety protocols should be followed when working with this compound. It is advisable to consult the safety data sheet (SDS) for specific information on potential hazards and recommended safety precautions.
Q14: Is there information available on the environmental impact of BGE?
A14: While dedicated studies on the environmental impact of BGE are limited, research on similar epoxy compounds suggests potential concerns. Responsible waste management and exploration of biodegradable alternatives are crucial to mitigate any negative environmental effects.
Q15: What analytical methods are used to quantify this compound?
A15: High-performance liquid chromatography (HPLC) is a common technique used to separate and quantify BGE enantiomers, often employing chiral stationary phases like Chiralcel OD [].
Q16: What factors can influence the HPLC separation of this compound enantiomers?
A16: The resolution and retention times of BGE enantiomers during HPLC analysis can be influenced by various factors, including the composition of the mobile phase (e.g., isopropyl alcohol content) and the column temperature [].
Disclaimer and Information on In-Vitro Research Products
Please be aware that all articles and product information presented on BenchChem are intended solely for informational purposes. The products available for purchase on BenchChem are specifically designed for in-vitro studies, which are conducted outside of living organisms. In-vitro studies, derived from the Latin term "in glass," involve experiments performed in controlled laboratory settings using cells or tissues. It is important to note that these products are not categorized as medicines or drugs, and they have not received approval from the FDA for the prevention, treatment, or cure of any medical condition, ailment, or disease. We must emphasize that any form of bodily introduction of these products into humans or animals is strictly prohibited by law. It is essential to adhere to these guidelines to ensure compliance with legal and ethical standards in research and experimentation.