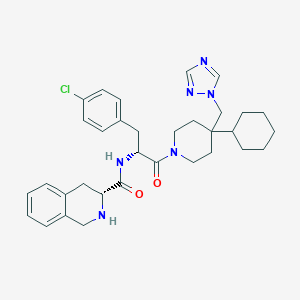
Thiq
Overview
Description
Tetrahydroisoquinoline is an organic compound with the chemical formula C₉H₁₁N. It is classified as a secondary amine and is derived from isoquinoline by hydrogenation. Tetrahydroisoquinoline is a colorless viscous liquid that is miscible with most organic solvents. The tetrahydroisoquinoline skeleton is encountered in a number of bioactive compounds and drugs .
Scientific Research Applications
Tetrahydroisoquinoline and its derivatives have diverse biological activities and are used in various fields:
Mechanism of Action
Target of Action
Tetrahydroisoquinoline (THIQ) is a secondary amine with the chemical formula C9H11N . This compound-based natural and synthetic compounds exert diverse biological activities against various infective pathogens and neurodegenerative disorders . This compound is known to interact with endorphin receptors and melanocortin receptor subtype MC4 . These receptors play crucial roles in various biological processes, including pain suppression and sexual activity .
Mode of Action
This compound acts as an agonist, stimulating the endorphin receptors . It is produced when monoamine neurotransmitters break down, and monoamine oxidase attacks the neurotransmitters, wiping out the receptors for recycling. When acetaldehyde is present, this compound forms, thereby plugging into the endorphin receptors and stimulating them . This interaction results in changes in the cellular activities associated with these receptors.
Biochemical Pathways
The biochemical pathways affected by this compound are primarily related to the neurotransmitter systems. This compound interacts with endorphin receptors, which are involved in pain suppression and other neurological functions . The exact downstream effects of this interaction can vary, but they generally involve changes in neuronal signaling and potentially the modulation of various physiological processes.
Pharmacokinetics
One study on a this compound derivative showed favorable in vitro adme properties . The compound exhibited potent inhibitory activity, high metabolic stability, and good permeability, suggesting a favorable bioavailability profile.
Result of Action
The molecular and cellular effects of this compound’s action are primarily related to its interaction with its target receptors. By acting as an agonist at endorphin receptors, this compound can modulate neuronal signaling and potentially influence various physiological processes . .
Biochemical Analysis
Biochemical Properties
THIQ interacts with various enzymes, proteins, and other biomolecules. It is known to amplify the effects of dopamine, a neurotransmitter associated with pleasure and reward . This interaction suggests that this compound may play a significant role in biochemical reactions related to mood regulation and reward response.
Cellular Effects
The effects of this compound on cells are complex and multifaceted. It influences cell function by acting on dopamine receptors, potentially impacting cell signaling pathways, gene expression, and cellular metabolism
Molecular Mechanism
At the molecular level, this compound exerts its effects through binding interactions with dopamine receptors . This binding can influence enzyme activity and gene expression, although the specifics of these interactions are still being explored.
Temporal Effects in Laboratory Settings
The effects of this compound can change over time in laboratory settings. While specific details about its stability, degradation, and long-term effects on cellular function are still being researched, it is known that this compound can have lasting impacts on the cells it interacts with .
Dosage Effects in Animal Models
The effects of this compound can vary with different dosages in animal models. While specific threshold effects and potential toxicities at high doses are still being studied, it is clear that dosage plays a significant role in the impact of this compound .
Metabolic Pathways
This compound is involved in several metabolic pathways, interacting with various enzymes and cofactors
Transport and Distribution
The transport and distribution of this compound within cells and tissues are complex processes that involve various transporters and binding proteins . The specifics of how this compound localization or accumulation is influenced by these factors are still being researched.
Subcellular Localization
The subcellular localization of this compound and its effects on activity or function are areas of active research. It is possible that specific targeting signals or post-translational modifications direct this compound to specific compartments or organelles .
Preparation Methods
Synthetic Routes and Reaction Conditions
One of the earliest methods for synthesizing tetrahydroisoquinoline was described by Pictet and Spengler in 1911. This method involves the reaction of phenylethylamine with dimethoxymethane in the presence of aqueous hydrochloric acid at 100°C, yielding tetrahydroisoquinoline in 40% yield . Later modifications replaced dimethoxymethane with aldehydes to produce substituted tetrahydroisoquinolines .
Industrial Production Methods
Industrial production of tetrahydroisoquinoline often involves catalytic hydrogenation of isoquinoline. This process typically uses a metal catalyst such as palladium or platinum under high pressure and temperature conditions to achieve the hydrogenation .
Chemical Reactions Analysis
Types of Reactions
Tetrahydroisoquinoline undergoes various types of chemical reactions, including:
Reduction: It can be further hydrogenated to produce decahydroisoquinoline.
Substitution: Tetrahydroisoquinoline can form salts with strong acids due to its weakly basic properties.
Common Reagents and Conditions
Oxidation: Hydrogen peroxide and selenium dioxide.
Reduction: Metal catalysts such as palladium or platinum.
Substitution: Strong acids like hydrochloric acid.
Major Products Formed
Oxidation: Corresponding nitrone.
Reduction: Decahydroisoquinoline.
Substitution: Salts of tetrahydroisoquinoline.
Comparison with Similar Compounds
Similar Compounds
Isoquinoline: The parent compound from which tetrahydroisoquinoline is derived.
Decahydroisoquinoline: A fully hydrogenated derivative of tetrahydroisoquinoline.
Norsalsolinol: A neurotoxic derivative of tetrahydroisoquinoline.
Uniqueness
Tetrahydroisoquinoline is unique due to its structural versatility and the wide range of biological activities exhibited by its derivatives. Its ability to form various bioactive compounds makes it a valuable scaffold in medicinal chemistry .
Properties
IUPAC Name |
(3R)-N-[(2R)-3-(4-chlorophenyl)-1-[4-cyclohexyl-4-(1,2,4-triazol-1-ylmethyl)piperidin-1-yl]-1-oxopropan-2-yl]-1,2,3,4-tetrahydroisoquinoline-3-carboxamide | |
---|---|---|
Source | PubChem | |
URL | https://pubchem.ncbi.nlm.nih.gov | |
Description | Data deposited in or computed by PubChem | |
InChI |
InChI=1S/C33H41ClN6O2/c34-28-12-10-24(11-13-28)18-30(38-31(41)29-19-25-6-4-5-7-26(25)20-36-29)32(42)39-16-14-33(15-17-39,21-40-23-35-22-37-40)27-8-2-1-3-9-27/h4-7,10-13,22-23,27,29-30,36H,1-3,8-9,14-21H2,(H,38,41)/t29-,30-/m1/s1 | |
Source | PubChem | |
URL | https://pubchem.ncbi.nlm.nih.gov | |
Description | Data deposited in or computed by PubChem | |
InChI Key |
HLCHESOMJVGDSJ-LOYHVIPDSA-N | |
Source | PubChem | |
URL | https://pubchem.ncbi.nlm.nih.gov | |
Description | Data deposited in or computed by PubChem | |
Canonical SMILES |
C1CCC(CC1)C2(CCN(CC2)C(=O)C(CC3=CC=C(C=C3)Cl)NC(=O)C4CC5=CC=CC=C5CN4)CN6C=NC=N6 | |
Source | PubChem | |
URL | https://pubchem.ncbi.nlm.nih.gov | |
Description | Data deposited in or computed by PubChem | |
Isomeric SMILES |
C1CCC(CC1)C2(CCN(CC2)C(=O)[C@@H](CC3=CC=C(C=C3)Cl)NC(=O)[C@H]4CC5=CC=CC=C5CN4)CN6C=NC=N6 | |
Source | PubChem | |
URL | https://pubchem.ncbi.nlm.nih.gov | |
Description | Data deposited in or computed by PubChem | |
Molecular Formula |
C33H41ClN6O2 | |
Source | PubChem | |
URL | https://pubchem.ncbi.nlm.nih.gov | |
Description | Data deposited in or computed by PubChem | |
DSSTOX Substance ID |
DTXSID00953320 | |
Record name | THIQ | |
Source | EPA DSSTox | |
URL | https://comptox.epa.gov/dashboard/DTXSID00953320 | |
Description | DSSTox provides a high quality public chemistry resource for supporting improved predictive toxicology. | |
Molecular Weight |
589.2 g/mol | |
Source | PubChem | |
URL | https://pubchem.ncbi.nlm.nih.gov | |
Description | Data deposited in or computed by PubChem | |
CAS No. |
312637-48-2 | |
Record name | (3R)-N-[(1R)-1-[(4-Chlorophenyl)methyl]-2-[4-cyclohexyl-4-(1H-1,2,4-triazol-1-ylmethyl)-1-piperidinyl]-2-oxoethyl]-1,2,3,4-tetrahydro-3-isoquinolinecarboxamide | |
Source | CAS Common Chemistry | |
URL | https://commonchemistry.cas.org/detail?cas_rn=312637-48-2 | |
Description | CAS Common Chemistry is an open community resource for accessing chemical information. Nearly 500,000 chemical substances from CAS REGISTRY cover areas of community interest, including common and frequently regulated chemicals, and those relevant to high school and undergraduate chemistry classes. This chemical information, curated by our expert scientists, is provided in alignment with our mission as a division of the American Chemical Society. | |
Explanation | The data from CAS Common Chemistry is provided under a CC-BY-NC 4.0 license, unless otherwise stated. | |
Record name | THIQ | |
Source | ChemIDplus | |
URL | https://pubchem.ncbi.nlm.nih.gov/substance/?source=chemidplus&sourceid=0312637482 | |
Description | ChemIDplus is a free, web search system that provides access to the structure and nomenclature authority files used for the identification of chemical substances cited in National Library of Medicine (NLM) databases, including the TOXNET system. | |
Record name | THIQ | |
Source | EPA DSSTox | |
URL | https://comptox.epa.gov/dashboard/DTXSID00953320 | |
Description | DSSTox provides a high quality public chemistry resource for supporting improved predictive toxicology. | |
Retrosynthesis Analysis
AI-Powered Synthesis Planning: Our tool employs the Template_relevance Pistachio, Template_relevance Bkms_metabolic, Template_relevance Pistachio_ringbreaker, Template_relevance Reaxys, Template_relevance Reaxys_biocatalysis model, leveraging a vast database of chemical reactions to predict feasible synthetic routes.
One-Step Synthesis Focus: Specifically designed for one-step synthesis, it provides concise and direct routes for your target compounds, streamlining the synthesis process.
Accurate Predictions: Utilizing the extensive PISTACHIO, BKMS_METABOLIC, PISTACHIO_RINGBREAKER, REAXYS, REAXYS_BIOCATALYSIS database, our tool offers high-accuracy predictions, reflecting the latest in chemical research and data.
Strategy Settings
Precursor scoring | Relevance Heuristic |
---|---|
Min. plausibility | 0.01 |
Model | Template_relevance |
Template Set | Pistachio/Bkms_metabolic/Pistachio_ringbreaker/Reaxys/Reaxys_biocatalysis |
Top-N result to add to graph | 6 |
Feasible Synthetic Routes
A: Tetrahydroisoquinoline (THIQ) derivatives exhibit a diverse range of interactions with biological targets. For example, they act as potent inhibitors of phenylethanolamine N-methyltransferase (PNMT), the enzyme responsible for epinephrine biosynthesis. [] This inhibition arises from the binding of THIQs to the PNMT active site, mimicking the natural substrate. [] The downstream effect of PNMT inhibition is a reduction in epinephrine levels, potentially impacting the sympathetic nervous system. []
ANone: Unfortunately, the provided research focuses primarily on the structure-activity relationships and biological effects of various this compound derivatives rather than detailing the specific spectroscopic data of the unsubstituted this compound molecule.
ANone: The provided research primarily focuses on the biological activity and structure-activity relationships of this compound derivatives and does not provide information regarding material compatibility, stability, or applications outside of a biological context.
A: Computational tools, particularly quantitative structure-activity relationship (QSAR) studies and comparative molecular field analysis (CoMFA), have been instrumental in understanding the interaction of THIQs with biological targets and driving the design of selective inhibitors. [] For instance, QSAR studies revealed distinct steric and electronic requirements for binding to PNMT and the α2-adrenoceptor, suggesting that subtle structural modifications could modulate selectivity. [] Furthermore, CoMFA models provided insights into the three-dimensional aspects of this compound binding, enabling the visualization of key interactions within the active sites. []
A: Extensive SAR studies have been conducted on this compound derivatives, revealing key structural features influencing their potency and selectivity for targets like PNMT and the α2-adrenoceptor. [] For instance, incorporating a 3-hydroxymethyl substituent on the this compound scaffold significantly enhances selectivity for PNMT over the α2-adrenoceptor compared to a 3-methyl substituent. [] This selectivity enhancement is attributed to the potential for hydrogen bond interactions between the hydroxymethyl group and the PNMT active site. []
ANone: The provided research predominantly emphasizes the biological activity and structure-activity relationships of this compound derivatives and does not delve into stability, formulation strategies, or methods to improve bioavailability.
ANone: The provided research papers primarily focus on the scientific and medicinal aspects of this compound derivatives, and do not discuss SHE regulations, compliance, risk minimization, or responsible practices related to their production or use.
A: While the provided research primarily focuses on in vitro studies of this compound derivatives, there is limited information available regarding their in vivo pharmacokinetic properties. One study investigated the blood-brain barrier (BBB) permeability of a series of THIQs. [] A correlation between lipophilicity (expressed as ClogP) and BBB permeability was observed, suggesting that THIQs with ClogP values exceeding 0.13-0.57 might exhibit some degree of brain penetration. []
A: The research provides evidence for the in vitro and in vivo efficacy of this compound derivatives, mainly focusing on their interaction with PNMT and the α2-adrenoceptor. [, , , , ] Cell-based assays, including radioligand binding studies and functional assays using cell lines expressing the target receptors, have been instrumental in assessing the affinity, selectivity, and functional effects of this compound analogs. [, , , , ]
A: While the provided text does not contain detailed toxicology studies, one study assessed the cytotoxicity of 1-benzyl-1,2,3,4-tetrahydroisoquinoline-6,7-diol (1-benz-6,7-diol this compound) using an in vitro 3D neurosphere model. [] The results indicate that 1-benz-6,7-diol-THIQ did not exhibit significant cytotoxicity or oxidative stress induction after 24 hours of exposure at various concentrations. []
A: The provided research primarily focuses on the synthesis and in vitro evaluation of this compound derivatives. While there is limited information about drug delivery and targeting strategies, one study demonstrated that incorporating a fluoromethyl group at the 3-position of the this compound scaffold could enhance blood-brain barrier penetration, suggesting potential for targeting central nervous system disorders. []
Disclaimer and Information on In-Vitro Research Products
Please be aware that all articles and product information presented on BenchChem are intended solely for informational purposes. The products available for purchase on BenchChem are specifically designed for in-vitro studies, which are conducted outside of living organisms. In-vitro studies, derived from the Latin term "in glass," involve experiments performed in controlled laboratory settings using cells or tissues. It is important to note that these products are not categorized as medicines or drugs, and they have not received approval from the FDA for the prevention, treatment, or cure of any medical condition, ailment, or disease. We must emphasize that any form of bodily introduction of these products into humans or animals is strictly prohibited by law. It is essential to adhere to these guidelines to ensure compliance with legal and ethical standards in research and experimentation.