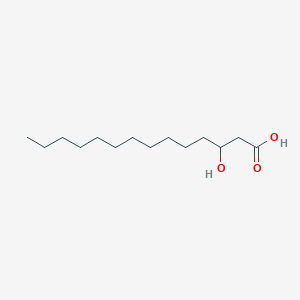
3-Hydroxytetradecanoic acid
Overview
Description
Scientific Research Applications
3-Hydroxytetradecanoic acid has numerous applications in scientific research:
Safety and Hazards
When handling 3-Hydroxytetradecanoic acid, it is advised to avoid dust formation, breathing mist, gas, or vapors, and contacting with skin and eye . It is recommended to use personal protective equipment, wear chemical impermeable gloves, ensure adequate ventilation, remove all sources of ignition, and evacuate personnel to safe areas .
Future Directions
Mechanism of Action
Target of Action
3-Hydroxytetradecanoic acid primarily targets the Protein TonB in Escherichia coli (strain K12), Lymphocyte antigen 96 , and Toll-like receptor 4 in humans . These targets play crucial roles in bacterial iron uptake and immune response, respectively.
Mode of Action
For instance, in E. coli, it may influence the function of Protein TonB, which is essential for the active transport of iron .
Biochemical Pathways
This compound plays an intermediate role in fatty acid biosynthesis . It is a component of lipid A, a part of lipopolysaccharides in the outer membrane of Gram-negative bacteria . Lipid A is responsible for most of the endotoxic activities of lipopolysaccharides .
Result of Action
It has been found to have antioxidant, antielastase, and antiurease activities . These activities suggest that the compound could have potential therapeutic applications.
Action Environment
The action, efficacy, and stability of this compound can be influenced by various environmental factors. For instance, the compound’s enzymatic synthesis by porcine pancreas lipase is affected by the weight ratio of substrate to lipase and the reaction time . Furthermore, the compound’s bioactivity may vary depending on the biological environment, such as the presence of other molecules and pH conditions .
Biochemical Analysis
Biochemical Properties
3-Hydroxytetradecanoic acid is involved in various biochemical reactions. It is a component of lipid A, a part of lipopolysaccharides in the outer membrane of Gram-negative bacteria . It is also used in endotoxin and lipid A research
Cellular Effects
It has been suggested that it plays a role in biological processes such as oxidative stress, inflammation, and insulin resistance
Molecular Mechanism
It is known to be involved in fatty acid biosynthesis
Temporal Effects in Laboratory Settings
It has been observed that the concentrations of 3-Hydroxy fatty acids in the supernatant follow bacterial growth . Information on the product’s stability, degradation, and long-term effects on cellular function observed in in vitro or in vivo studies is limited.
Metabolic Pathways
Preparation Methods
Synthetic Routes and Reaction Conditions
3-Hydroxytetradecanoic acid can be synthesized through various methods. One common approach involves the enantioselective synthesis using porcine pancreas lipase to catalyze the hydrolysis of racemic methyl 3-hydroxytetradecanoate in an aqueous medium . This method allows for the production of optically pure R and S enantiomers of this compound. The reaction conditions include a substrate/lipase weight ratio of 3:1 and an incubation time of 7 hours, resulting in a high enantiomeric excess of greater than 99% .
Industrial Production Methods
Industrial production of this compound often involves microbial fermentation processes. Certain bacteria, such as Malassezia furfur, can metabolize substrates to produce this compound . This biotechnological approach is environmentally friendly and can be scaled up for large-scale production.
Chemical Reactions Analysis
Types of Reactions
3-Hydroxytetradecanoic acid undergoes various chemical reactions, including:
Oxidation: The hydroxyl group can be oxidized to form a ketone or carboxylic acid.
Reduction: The carboxyl group can be reduced to form an alcohol.
Esterification: The hydroxyl group can react with carboxylic acids to form esters.
Substitution: The hydroxyl group can be substituted with other functional groups.
Common Reagents and Conditions
Oxidation: Common oxidizing agents include potassium permanganate and chromium trioxide.
Reduction: Reducing agents such as lithium aluminum hydride or sodium borohydride are used.
Esterification: Acid catalysts like sulfuric acid or p-toluenesulfonic acid are typically employed.
Substitution: Reagents such as thionyl chloride or phosphorus tribromide can be used for substitution reactions.
Major Products
Oxidation: Produces 3-ketotetradecanoic acid or tetradecanedioic acid.
Reduction: Produces 3-hydroxytetradecanol.
Esterification: Produces esters like methyl 3-hydroxytetradecanoate.
Substitution: Produces various substituted derivatives depending on the reagent used.
Comparison with Similar Compounds
3-Hydroxytetradecanoic acid can be compared with other similar compounds, such as:
3-Hydroxybutyric acid: A short-chain hydroxy fatty acid involved in ketone body metabolism.
3-Hydroxyvaleric acid: A medium-chain hydroxy fatty acid used in the synthesis of biodegradable polymers.
3-Hydroxyhexanoic acid: A medium-chain hydroxy fatty acid with applications in bioplastics production.
3-Hydroxyoctanoic acid: A medium-chain hydroxy fatty acid used in the synthesis of fine chemicals.
Properties
IUPAC Name |
3-hydroxytetradecanoic acid | |
---|---|---|
Source | PubChem | |
URL | https://pubchem.ncbi.nlm.nih.gov | |
Description | Data deposited in or computed by PubChem | |
InChI |
InChI=1S/C14H28O3/c1-2-3-4-5-6-7-8-9-10-11-13(15)12-14(16)17/h13,15H,2-12H2,1H3,(H,16,17) | |
Source | PubChem | |
URL | https://pubchem.ncbi.nlm.nih.gov | |
Description | Data deposited in or computed by PubChem | |
InChI Key |
ATRNZOYKSNPPBF-UHFFFAOYSA-N | |
Source | PubChem | |
URL | https://pubchem.ncbi.nlm.nih.gov | |
Description | Data deposited in or computed by PubChem | |
Canonical SMILES |
CCCCCCCCCCCC(CC(=O)O)O | |
Source | PubChem | |
URL | https://pubchem.ncbi.nlm.nih.gov | |
Description | Data deposited in or computed by PubChem | |
Molecular Formula |
C14H28O3 | |
Source | PubChem | |
URL | https://pubchem.ncbi.nlm.nih.gov | |
Description | Data deposited in or computed by PubChem | |
DSSTOX Substance ID |
DTXSID20941380 | |
Record name | 3-Hydroxytetradecanoic acid | |
Source | EPA DSSTox | |
URL | https://comptox.epa.gov/dashboard/DTXSID20941380 | |
Description | DSSTox provides a high quality public chemistry resource for supporting improved predictive toxicology. | |
Molecular Weight |
244.37 g/mol | |
Source | PubChem | |
URL | https://pubchem.ncbi.nlm.nih.gov | |
Description | Data deposited in or computed by PubChem | |
CAS No. |
1961-72-4 | |
Record name | (±)-3-Hydroxytetradecanoic acid | |
Source | CAS Common Chemistry | |
URL | https://commonchemistry.cas.org/detail?cas_rn=1961-72-4 | |
Description | CAS Common Chemistry is an open community resource for accessing chemical information. Nearly 500,000 chemical substances from CAS REGISTRY cover areas of community interest, including common and frequently regulated chemicals, and those relevant to high school and undergraduate chemistry classes. This chemical information, curated by our expert scientists, is provided in alignment with our mission as a division of the American Chemical Society. | |
Explanation | The data from CAS Common Chemistry is provided under a CC-BY-NC 4.0 license, unless otherwise stated. | |
Record name | beta-Hydroxymyristic acid | |
Source | ChemIDplus | |
URL | https://pubchem.ncbi.nlm.nih.gov/substance/?source=chemidplus&sourceid=0001961724 | |
Description | ChemIDplus is a free, web search system that provides access to the structure and nomenclature authority files used for the identification of chemical substances cited in National Library of Medicine (NLM) databases, including the TOXNET system. | |
Record name | 3-Hydroxytetradecanoic acid | |
Source | EPA DSSTox | |
URL | https://comptox.epa.gov/dashboard/DTXSID20941380 | |
Description | DSSTox provides a high quality public chemistry resource for supporting improved predictive toxicology. | |
Record name | 3-HYDROXYMYRISTIC ACID, (±)- | |
Source | FDA Global Substance Registration System (GSRS) | |
URL | https://gsrs.ncats.nih.gov/ginas/app/beta/substances/FL8S7F2JJQ | |
Description | The FDA Global Substance Registration System (GSRS) enables the efficient and accurate exchange of information on what substances are in regulated products. Instead of relying on names, which vary across regulatory domains, countries, and regions, the GSRS knowledge base makes it possible for substances to be defined by standardized, scientific descriptions. | |
Explanation | Unless otherwise noted, the contents of the FDA website (www.fda.gov), both text and graphics, are not copyrighted. They are in the public domain and may be republished, reprinted and otherwise used freely by anyone without the need to obtain permission from FDA. Credit to the U.S. Food and Drug Administration as the source is appreciated but not required. | |
Retrosynthesis Analysis
AI-Powered Synthesis Planning: Our tool employs the Template_relevance Pistachio, Template_relevance Bkms_metabolic, Template_relevance Pistachio_ringbreaker, Template_relevance Reaxys, Template_relevance Reaxys_biocatalysis model, leveraging a vast database of chemical reactions to predict feasible synthetic routes.
One-Step Synthesis Focus: Specifically designed for one-step synthesis, it provides concise and direct routes for your target compounds, streamlining the synthesis process.
Accurate Predictions: Utilizing the extensive PISTACHIO, BKMS_METABOLIC, PISTACHIO_RINGBREAKER, REAXYS, REAXYS_BIOCATALYSIS database, our tool offers high-accuracy predictions, reflecting the latest in chemical research and data.
Strategy Settings
Precursor scoring | Relevance Heuristic |
---|---|
Min. plausibility | 0.01 |
Model | Template_relevance |
Template Set | Pistachio/Bkms_metabolic/Pistachio_ringbreaker/Reaxys/Reaxys_biocatalysis |
Top-N result to add to graph | 6 |
Feasible Synthetic Routes
Disclaimer and Information on In-Vitro Research Products
Please be aware that all articles and product information presented on BenchChem are intended solely for informational purposes. The products available for purchase on BenchChem are specifically designed for in-vitro studies, which are conducted outside of living organisms. In-vitro studies, derived from the Latin term "in glass," involve experiments performed in controlled laboratory settings using cells or tissues. It is important to note that these products are not categorized as medicines or drugs, and they have not received approval from the FDA for the prevention, treatment, or cure of any medical condition, ailment, or disease. We must emphasize that any form of bodily introduction of these products into humans or animals is strictly prohibited by law. It is essential to adhere to these guidelines to ensure compliance with legal and ethical standards in research and experimentation.