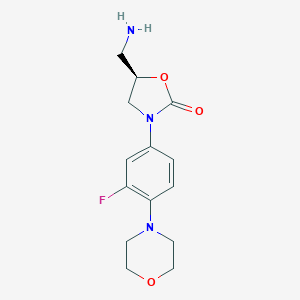
Deacetyl (R)-Linezolid
Overview
Description
Deacetyl ®-Linezolid is a derivative of Linezolid, an antibiotic used to treat infections caused by Gram-positive bacteria
Preparation Methods
Synthetic Routes and Reaction Conditions
The synthesis of Deacetyl ®-Linezolid involves the deacetylation of Linezolid. This can be achieved through various chemical reactions, including hydrolysis and enzymatic processes. The reaction conditions typically involve the use of a base, such as sodium hydroxide, in an aqueous or organic solvent. The reaction is carried out at elevated temperatures to facilitate the removal of the acetyl group.
Industrial Production Methods
In an industrial setting, the production of Deacetyl ®-Linezolid can be scaled up using continuous flow reactors. This allows for the efficient and controlled deacetylation of Linezolid. The process involves the use of high-purity reagents and stringent quality control measures to ensure the consistency and purity of the final product.
Chemical Reactions Analysis
Types of Reactions
Deacetyl ®-Linezolid undergoes various chemical reactions, including:
Oxidation: The compound can be oxidized to form corresponding oxides.
Reduction: Reduction reactions can convert Deacetyl ®-Linezolid into different reduced forms.
Substitution: The compound can undergo substitution reactions where functional groups are replaced by other groups.
Common Reagents and Conditions
Oxidation: Common oxidizing agents include hydrogen peroxide and potassium permanganate.
Reduction: Reducing agents such as sodium borohydride and lithium aluminum hydride are used.
Substitution: Reagents like halogens and alkylating agents are employed in substitution reactions.
Major Products Formed
The major products formed from these reactions depend on the specific conditions and reagents used. For example, oxidation may yield oxides, while reduction can produce various reduced derivatives.
Scientific Research Applications
Deacetyl ®-Linezolid has several scientific research applications, including:
Chemistry: Used as a precursor in the synthesis of more complex molecules.
Biology: Studied for its potential effects on bacterial growth and resistance mechanisms.
Medicine: Investigated for its potential use as an antibiotic with improved efficacy and reduced side effects.
Industry: Utilized in the development of new materials and chemical processes.
Mechanism of Action
The mechanism of action of Deacetyl ®-Linezolid involves the inhibition of bacterial protein synthesis. It binds to the bacterial ribosome, preventing the formation of the initiation complex for protein synthesis. This action disrupts the production of essential proteins, leading to the death of the bacterial cell. The molecular targets include the 23S ribosomal RNA of the 50S subunit of the bacterial ribosome.
Comparison with Similar Compounds
Similar Compounds
Linezolid: The parent compound from which Deacetyl ®-Linezolid is derived.
Tedizolid: Another oxazolidinone antibiotic with similar mechanisms of action.
Radezolid: A newer oxazolidinone with enhanced activity against resistant strains.
Uniqueness
Deacetyl ®-Linezolid is unique due to its deacetylated structure, which may confer different pharmacokinetic and pharmacodynamic properties compared to its parent compound, Linezolid. This structural difference can potentially lead to improved efficacy, reduced resistance, and different side effect profiles.
Properties
IUPAC Name |
(5R)-5-(aminomethyl)-3-(3-fluoro-4-morpholin-4-ylphenyl)-1,3-oxazolidin-2-one | |
---|---|---|
Source | PubChem | |
URL | https://pubchem.ncbi.nlm.nih.gov | |
Description | Data deposited in or computed by PubChem | |
InChI |
InChI=1S/C14H18FN3O3/c15-12-7-10(18-9-11(8-16)21-14(18)19)1-2-13(12)17-3-5-20-6-4-17/h1-2,7,11H,3-6,8-9,16H2/t11-/m1/s1 | |
Source | PubChem | |
URL | https://pubchem.ncbi.nlm.nih.gov | |
Description | Data deposited in or computed by PubChem | |
InChI Key |
VXIWZOWWQMRVRF-LLVKDONJSA-N | |
Source | PubChem | |
URL | https://pubchem.ncbi.nlm.nih.gov | |
Description | Data deposited in or computed by PubChem | |
Canonical SMILES |
C1COCCN1C2=C(C=C(C=C2)N3CC(OC3=O)CN)F | |
Source | PubChem | |
URL | https://pubchem.ncbi.nlm.nih.gov | |
Description | Data deposited in or computed by PubChem | |
Isomeric SMILES |
C1COCCN1C2=C(C=C(C=C2)N3C[C@H](OC3=O)CN)F | |
Source | PubChem | |
URL | https://pubchem.ncbi.nlm.nih.gov | |
Description | Data deposited in or computed by PubChem | |
Molecular Formula |
C14H18FN3O3 | |
Source | PubChem | |
URL | https://pubchem.ncbi.nlm.nih.gov | |
Description | Data deposited in or computed by PubChem | |
Molecular Weight |
295.31 g/mol | |
Source | PubChem | |
URL | https://pubchem.ncbi.nlm.nih.gov | |
Description | Data deposited in or computed by PubChem | |
Synthesis routes and methods
Procedure details
Disclaimer and Information on In-Vitro Research Products
Please be aware that all articles and product information presented on BenchChem are intended solely for informational purposes. The products available for purchase on BenchChem are specifically designed for in-vitro studies, which are conducted outside of living organisms. In-vitro studies, derived from the Latin term "in glass," involve experiments performed in controlled laboratory settings using cells or tissues. It is important to note that these products are not categorized as medicines or drugs, and they have not received approval from the FDA for the prevention, treatment, or cure of any medical condition, ailment, or disease. We must emphasize that any form of bodily introduction of these products into humans or animals is strictly prohibited by law. It is essential to adhere to these guidelines to ensure compliance with legal and ethical standards in research and experimentation.