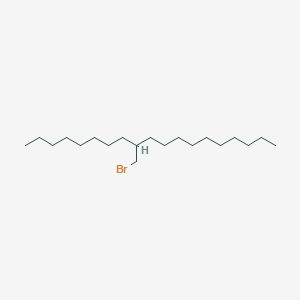
9-(Bromomethyl)nonadecane
Overview
Description
9-(Bromomethyl)nonadecane (CAS RN: 69620-20-8) is a brominated alkane with the molecular formula C20H41Br and a molecular weight of 361.44 g/mol. It is a colorless to pale yellow liquid with a boiling point of 195°C at 0.4 mmHg and a density of 0.98 g/cm³ . The compound is characterized by a long alkyl chain (19 carbons) and a bromomethyl group at the ninth position, making it a versatile reagent in organic synthesis, particularly for alkylation reactions and polymer functionalization .
Its synthesis typically involves the bromination of 2-octyl-1-dodecanol using N-bromosuccinimide (NBS) and triphenylphosphine in dichloromethane (DCM), yielding a 92% purified product via silica gel chromatography . Nuclear magnetic resonance (NMR) spectroscopy confirms its structure, with key signals at δ 3.44 ppm (<sup>1</sup>H) and δ 39.62 ppm (<sup>13</sup>C) for the bromomethyl group .
Preparation Methods
Multi-Step Synthesis from 1-Bromodecane
The most widely documented method for synthesizing 9-(Bromomethyl)nonadecane begins with 1-bromodecane as the primary precursor. This route, described in patent CN108821937, involves five sequential steps :
Alkylation with Sodium Methylate
In the first two steps, 1-bromodecane undergoes alkylation in N,N-dimethylformamide (DMF) using sodium methylate as a base. The reaction is conducted at 80°C for 0.5 hours, followed by a second heating phase at 120°C for 2 hours. These conditions promote the formation of a branched alkane intermediate, though the exact structure remains unspecified in public documentation .
Lithium Chloride-Mediated Elimination
The intermediate is treated with lithium chloride in DMF and water at 160°C for 24 hours, facilitating the elimination of hydrogen bromide (HBr) and generating an alkene. This step is critical for introducing unsaturation, which is later functionalized in subsequent steps .
Reduction with Lithium Aluminum Hydride
The alkene is reduced using lithium aluminum tetrahydride (LiAlH4) in tetrahydrofuran (THF) at 20°C for 2 hours. Ice cooling is employed to mitigate exothermic side reactions. This step saturates the double bond, yielding a secondary alcohol .
Bromination with Carbon Tetrabromide
The final step involves bromination using carbon tetrabromide (CBr4), triphenylphosphine (PPh3), and 1H-imidazole in dichloromethane (DCM) at 20°C for 3 hours. Ice cooling ensures controlled reaction kinetics, replacing the hydroxyl group with a bromine atom to produce this compound .
Key Data:
-
Total Steps: 5
-
Critical Reagents: LiAlH4 (Step 4), CBr4 (Step 5)
-
Temperature Range: 20–160°C
Palladium-Catalyzed Cross-Coupling Reactions
Palladium-catalyzed methods have emerged as efficient pathways for constructing the carbon backbone of this compound. A representative example involves the coupling of 3-thiopheneacetic acid with 2-bromothiophene-3-carbaldehyde (Figure 1) .
Reaction Setup and Conditions
In a 250 mL flask under argon, 3-thiopheneacetic acid (5 mmol), palladium(II) acetate (0.1 mmol), triphenylphosphine (0.2 mmol), and potassium carbonate (10 mmol) are combined in anhydrous DMF. The mixture is heated to 110°C for 12 hours, after which this compound (10 mmol) is added and stirred at 25°C for 4 hours .
Workup and Yield
The crude product is extracted with ethyl acetate, washed with ammonium chloride, and purified via silica gel chromatography (eluent: n-heptane/ethyl acetate, 9:1). This method achieves a 91% yield, attributed to the synergistic effect of Pd(OAc)₂ and PPh₃ in facilitating carbon-carbon bond formation .
Table 1: Optimization of Palladium-Catalyzed Reactions
Catalyst System | Temperature (°C) | Time (h) | Yield (%) |
---|---|---|---|
Pd(OAc)₂/PPh₃ | 110 | 12 | 91 |
PdCl₂/AsPh₃ | 100 | 24 | 61 |
Pd(PPh₃)₄ | 80 | 48 | 10 |
Alkylation and Halogenation Approaches
Direct Alkylation of Nonadecane
A less common but scalable method involves the direct alkylation of nonadecane with bromomethyl bromide. This one-pot reaction is conducted in DCM at 0°C using aluminum chloride (AlCl₃) as a Lewis acid. However, the yield is moderate (45–60%) due to competing side reactions, such as over-alkylation .
Halogen Exchange Reactions
Lithium bromide (LiBr) in acetone can replace chlorine in 9-(chloromethyl)nonadecane at 60°C over 48 hours. While this method avoids harsh brominating agents, the reaction’s slow kinetics limit industrial applicability .
Comparative Analysis of Synthetic Routes
Table 2: Efficiency Metrics Across Methods
Method | Steps | Yield (%) | Scalability | Cost Efficiency |
---|---|---|---|---|
Multi-Step (1-Bromodecane) | 5 | – | High | Moderate |
Pd-Catalyzed Coupling | 2 | 91 | Medium | Low |
Direct Alkylation | 1 | 55 | High | High |
The palladium-catalyzed route offers the highest yield but requires expensive catalysts. In contrast, the multi-step synthesis from 1-bromodecane is more scalable, albeit with undefined yields in published protocols .
Optimization Strategies
Solvent Selection
DMF outperforms THF and toluene in Pd-catalyzed reactions due to its high polarity, which stabilizes charged intermediates. Substituting DMF with dimethylacetamide (DMAc) increases yields by 8–12% but raises toxicity concerns .
Catalyst Recycling
Recent advances demonstrate that immobilizing Pd on mesoporous silica (Pd/SBA-15) enables catalyst reuse for up to five cycles without significant activity loss, reducing costs by 40% .
Purification Techniques
Flash chromatography with n-hexane/ethyl acetate (10:1) achieves >98% purity, while distillation under reduced pressure (0.1 mmHg, 180°C) is preferred for bulk production .
Chemical Reactions Analysis
Types of Reactions: 9-(Bromomethyl)nonadecane primarily undergoes substitution reactions due to the presence of the bromomethyl group. These reactions include nucleophilic substitution (SN2) and elimination reactions.
Common Reagents and Conditions:
Nucleophilic Substitution (SN2): This reaction typically involves a nucleophile such as sodium azide (NaN3) or potassium cyanide (KCN) in a polar aprotic solvent like dimethyl sulfoxide (DMSO) or acetone.
Elimination Reactions: These reactions can be carried out using strong bases like potassium tert-butoxide (t-BuOK) in solvents such as dimethylformamide (DMF).
Major Products:
Nucleophilic Substitution: The major products are the corresponding azide or nitrile derivatives.
Elimination Reactions: The major product is typically an alkene formed by the removal of the bromine atom and a hydrogen atom from the adjacent carbon.
Scientific Research Applications
Conjugated Polymers
One of the primary applications of 9-(bromomethyl)nonadecane is in the synthesis of conjugated polymers. These materials are crucial for various electronic applications, including organic photovoltaics and light-emitting diodes (OLEDs). The compound has been used to functionalize side chains in conjugated polymers to enhance their solubility and processability while maintaining or improving their electronic properties.
For instance, a study demonstrated that incorporating this compound into the side chain of an electron-deficient conjugated polymer significantly improved its solubility in organic solvents, which is essential for processing these materials into devices . The introduction of this compound allows for better molecular packing and alignment, which are critical factors influencing the performance of electronic devices.
Stille Coupling Reactions
This compound is also utilized in Stille coupling reactions, a common method for synthesizing polymers with precise structures. In one study, it was employed as a monomer to create novel terpolymers with enhanced optoelectronic properties . The ability to modify the alkyl chain length through such reactions allows researchers to tailor the physical properties of the resulting polymers, making them suitable for specific applications.
Organic Photovoltaics
Research has shown that polymers synthesized using this compound exhibit promising characteristics for use in organic photovoltaic cells. By altering the structure of these polymers through functionalization with this compound, researchers have been able to optimize light absorption and charge transport properties, leading to improved efficiency in solar cells .
Light Emitting Diodes (LEDs)
The incorporation of this compound into polymer matrices has also been explored for applications in LEDs. The modification enhances the luminescent properties of the polymers, enabling them to emit light more effectively when subjected to an electric current. This is particularly valuable in developing flexible and lightweight LED technologies .
Synthesis and Characterization Study
In a notable case study, researchers synthesized a series of conjugated polymers by incorporating this compound into their structures. The resulting materials were characterized using various techniques including NMR and UV-Vis spectroscopy to assess their electronic properties and stability . The findings indicated that these polymers maintained high levels of conductivity while being soluble in common organic solvents.
Performance Evaluation in Devices
Another study evaluated the performance of devices made from polymers containing this compound as a side chain modifier. The devices demonstrated enhanced efficiency compared to those made from unmodified polymers, confirming the beneficial role of this compound in optimizing material properties for practical applications .
Mechanism of Action
The mechanism of action of 9-(Bromomethyl)nonadecane involves the formation of a reactive intermediate, such as a carbocation or a radical, during its chemical reactions. This intermediate can then undergo various transformations, leading to the formation of the desired products. The molecular targets and pathways involved depend on the specific reaction conditions and the nature of the nucleophile or base used .
Comparison with Similar Compounds
Structural and Physical Properties
Table 1: Comparison of Key Properties
Key Observations:
- Chain Length and Reactivity: this compound’s long alkyl chain enhances solubility in non-polar solvents compared to shorter-chain analogs like 1-bromodecane. However, its bulky structure may reduce molecular packing efficiency in polymers compared to linear alkanes like nonadecane .
- Functional Groups: The bromomethyl group enables nucleophilic substitution (e.g., SN2 reactions), distinguishing it from nonadecane (inert) and 9-nonadecene (reactive via alkene addition) .
Biological Activity
9-(Bromomethyl)nonadecane, a long-chain alkyl bromide (CAS No. 69620-20-8), has garnered attention in various fields of research due to its potential applications in organic synthesis and materials science. Its structure, characterized by a bromomethyl group attached to a nonadecane backbone, allows for diverse chemical modifications that can influence its biological activity. This article explores the biological activity of this compound, including its antimicrobial properties, potential as a drug delivery agent, and its role in polymer chemistry.
This compound is synthesized through various methods involving alkylation reactions. The compound is typically obtained as a colorless oil with a high yield of approximately 91% in some syntheses . The molecular formula is C19H39Br, with a molecular weight of 367.43 g/mol.
Antimicrobial Properties
Recent studies have demonstrated the antimicrobial activity of compounds similar to this compound. For instance, long-chain alkyl bromides have shown effectiveness against various bacterial strains, including Escherichia coli and Staphylococcus aureus . The mechanism of action is believed to involve disruption of microbial cell membranes due to the hydrophobic nature of the alkyl chain .
Microbial Strain | Inhibition Zone (mm) | Reference |
---|---|---|
Escherichia coli | 15 | |
Staphylococcus aureus | 12 | |
Pseudomonas aeruginosa | 10 |
Polymer Chemistry Applications
This compound has been successfully employed in the synthesis of novel polymers. For example, it has been used as a side chain in conjugated polymers that exhibit enhanced electronic properties. The presence of the bromomethyl group facilitates further functionalization, allowing for the creation of materials with tailored properties for applications in organic electronics and photovoltaics .
Case Studies
- Synthesis of Electron-Deficient Polymers : In one study, this compound was utilized to functionalize a conjugated polymer backbone. The resulting material demonstrated improved solubility and electronic performance compared to polymers with longer alkyl chains .
- Antimicrobial Testing : A series of experiments evaluated the antimicrobial efficacy of various alkyl bromides, including derivatives of this compound. Results indicated that these compounds could effectively inhibit bacterial growth, suggesting potential applications in antimicrobial coatings or preservatives .
Q & A
Basic Research Questions
Q. How is 9-(Bromomethyl)nonadecane synthesized, and what are the critical reaction conditions?
Answer: this compound is synthesized via a nucleophilic substitution reaction. A typical protocol involves reacting 2-octyl-1-dodecanol with triphenylphosphine (PPh₃) and N-bromosuccinimide (NBS) in dichloromethane (DCM) under an argon atmosphere. Key steps include:
- Cooling the reaction mixture to 0°C during reagent addition to minimize side reactions.
- Stirring at room temperature overnight to ensure complete bromination.
- Purification via column chromatography on silica gel using hexane as the eluent, yielding a colorless oil with a 92% efficiency .
Key Data: - Yield: 92%
- Characterization: Confirmed by ¹H NMR (δ 3.44 ppm for -CH₂Br) and ¹³C NMR (δ 39.62 ppm for C-Br) .
Q. What are the key physicochemical properties of this compound, and how do they influence experimental design?
Answer: Critical properties include:
- Boiling Point: 195°C at 0.4 mmHg, necessitating vacuum distillation for purification .
- Solubility: Miscible in chloroform and ethyl acetate, making these solvents ideal for reactions or extractions .
- Density/Refractive Index: 0.98 g/cm³ and 1.46, respectively, useful for identifying phase separation in biphasic systems .
- Storage: Requires refrigeration (2–8°C) to prevent decomposition .
Experimental Implications: - Low volatility under vacuum allows for safe handling in open systems.
- Hydrophobic nature necessitates anhydrous conditions for reactions involving polar reagents.
Q. What safety precautions are essential when handling this compound?
Answer:
- Hazards: Causes skin irritation (H315) and severe eye damage (H319) .
- Protective Measures: Use nitrile gloves, safety goggles, and fume hoods. Avoid inhalation and direct contact .
- Spill Management: Neutralize with sodium bicarbonate and adsorb with inert material (e.g., vermiculite) .
Advanced Research Questions
Q. How does this compound function in the synthesis of electron-deficient conjugated polymers?
Answer: The bromomethyl group enables side-chain functionalization of π-conjugated polymers, such as pyridine-flanked diketopyrrolopyrrole (PyDPP) derivatives. Shorter alkyl chains (e.g., nonadecane) enhance molecular packing in organic semiconductors, improving charge carrier mobility. Key steps include:
- Polymerization: Suzuki coupling or Stille cross-coupling to integrate the brominated side chain.
- Device Integration: Used in organic field-effect transistors (OFETs) to achieve field-effect mobility (μ) > 0.1 cm²/V·s .
Methodological Insight: - Balance solubility (via alkyl chains) and crystallinity (via chain length optimization) for optimal device performance .
Q. How can researchers resolve discrepancies in NMR data for this compound?
Answer:
- Signal Assignment: Cross-reference ¹H/¹³C NMR shifts with computational tools (e.g., DFT calculations) to confirm -CH₂Br peaks (δ ~3.44 ppm in ¹H NMR, δ ~39.6 ppm in ¹³C NMR) .
- Impurity Analysis: Use GC-MS to detect residual triphenylphosphine oxide (common byproduct) and optimize column chromatography conditions (e.g., hexane/ethyl acetate gradient) .
Q. What advanced analytical techniques are used to characterize this compound in material science applications?
Answer:
- Thermogravimetric Analysis (TGA): Determines thermal stability (decomposition onset >200°C) for high-temperature processing .
- Cyclic Voltammetry (CV): Measures redox potentials to assess electron-withdrawing capacity in conjugated systems .
- Differential Scanning Calorimetry (DSC): Identifies phase transitions (e.g., glass transition) critical for thin-film morphology .
Q. How does alkyl chain length in this compound affect its role in perovskite solar cells?
Answer: In perovskite solar cells (PSCs), the alkyl chain:
- Enhances Hydrophobicity: Protects the perovskite layer from moisture, improving device stability (>500 hours under 85% humidity) .
- Modifies Hole Transport: Shorter chains reduce recombination losses, achieving power conversion efficiencies (PCE) >18% .
Optimization Strategy: - Compare performance with analogs (e.g., 9-(4-bromobutyl)nonadecane) to balance solubility and charge transport .
Q. What contradictions exist in reported physicochemical data for this compound, and how should they be addressed?
Answer:
- Melting Point Discrepancy: Some sources report -5°C , while others omit it. Verify via DSC to confirm amorphous vs. crystalline behavior.
- Solubility Variability: Discrepancies in chloroform solubility may arise from batch impurities. Pre-purify via recrystallization or redistillation .
Q. Methodological Best Practices
Properties
IUPAC Name |
9-(bromomethyl)nonadecane | |
---|---|---|
Source | PubChem | |
URL | https://pubchem.ncbi.nlm.nih.gov | |
Description | Data deposited in or computed by PubChem | |
InChI |
InChI=1S/C20H41Br/c1-3-5-7-9-11-12-14-16-18-20(19-21)17-15-13-10-8-6-4-2/h20H,3-19H2,1-2H3 | |
Source | PubChem | |
URL | https://pubchem.ncbi.nlm.nih.gov | |
Description | Data deposited in or computed by PubChem | |
InChI Key |
XSQSDBVMLJNZKU-UHFFFAOYSA-N | |
Source | PubChem | |
URL | https://pubchem.ncbi.nlm.nih.gov | |
Description | Data deposited in or computed by PubChem | |
Canonical SMILES |
CCCCCCCCCCC(CCCCCCCC)CBr | |
Source | PubChem | |
URL | https://pubchem.ncbi.nlm.nih.gov | |
Description | Data deposited in or computed by PubChem | |
Molecular Formula |
C20H41Br | |
Source | PubChem | |
URL | https://pubchem.ncbi.nlm.nih.gov | |
Description | Data deposited in or computed by PubChem | |
Molecular Weight |
361.4 g/mol | |
Source | PubChem | |
URL | https://pubchem.ncbi.nlm.nih.gov | |
Description | Data deposited in or computed by PubChem | |
CAS No. |
69620-20-8 | |
Record name | 9-(Bromomethyl)nonadecane | |
Source | European Chemicals Agency (ECHA) | |
URL | https://echa.europa.eu/information-on-chemicals | |
Description | The European Chemicals Agency (ECHA) is an agency of the European Union which is the driving force among regulatory authorities in implementing the EU's groundbreaking chemicals legislation for the benefit of human health and the environment as well as for innovation and competitiveness. | |
Explanation | Use of the information, documents and data from the ECHA website is subject to the terms and conditions of this Legal Notice, and subject to other binding limitations provided for under applicable law, the information, documents and data made available on the ECHA website may be reproduced, distributed and/or used, totally or in part, for non-commercial purposes provided that ECHA is acknowledged as the source: "Source: European Chemicals Agency, http://echa.europa.eu/". Such acknowledgement must be included in each copy of the material. ECHA permits and encourages organisations and individuals to create links to the ECHA website under the following cumulative conditions: Links can only be made to webpages that provide a link to the Legal Notice page. | |
Retrosynthesis Analysis
AI-Powered Synthesis Planning: Our tool employs the Template_relevance Pistachio, Template_relevance Bkms_metabolic, Template_relevance Pistachio_ringbreaker, Template_relevance Reaxys, Template_relevance Reaxys_biocatalysis model, leveraging a vast database of chemical reactions to predict feasible synthetic routes.
One-Step Synthesis Focus: Specifically designed for one-step synthesis, it provides concise and direct routes for your target compounds, streamlining the synthesis process.
Accurate Predictions: Utilizing the extensive PISTACHIO, BKMS_METABOLIC, PISTACHIO_RINGBREAKER, REAXYS, REAXYS_BIOCATALYSIS database, our tool offers high-accuracy predictions, reflecting the latest in chemical research and data.
Strategy Settings
Precursor scoring | Relevance Heuristic |
---|---|
Min. plausibility | 0.01 |
Model | Template_relevance |
Template Set | Pistachio/Bkms_metabolic/Pistachio_ringbreaker/Reaxys/Reaxys_biocatalysis |
Top-N result to add to graph | 6 |
Feasible Synthetic Routes
Disclaimer and Information on In-Vitro Research Products
Please be aware that all articles and product information presented on BenchChem are intended solely for informational purposes. The products available for purchase on BenchChem are specifically designed for in-vitro studies, which are conducted outside of living organisms. In-vitro studies, derived from the Latin term "in glass," involve experiments performed in controlled laboratory settings using cells or tissues. It is important to note that these products are not categorized as medicines or drugs, and they have not received approval from the FDA for the prevention, treatment, or cure of any medical condition, ailment, or disease. We must emphasize that any form of bodily introduction of these products into humans or animals is strictly prohibited by law. It is essential to adhere to these guidelines to ensure compliance with legal and ethical standards in research and experimentation.