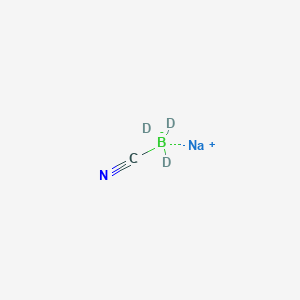
Sodium cyanoborodeuteride
Overview
Description
Sodium cyanoborodeuteride is a chemical compound with the formula NaBD₃CN. It is a deuterated derivative of sodium cyanoborohydride, where the hydrogen atoms are replaced by deuterium. This compound is widely used in organic synthesis, particularly for its mild reducing properties. It is a colorless salt that plays a crucial role in various chemical reactions, especially in the reduction of imines and carbonyl compounds .
Preparation Methods
Synthetic Routes and Reaction Conditions: Sodium cyanoborodeuteride is typically prepared by the deuteration of sodium cyanoborohydride. The process involves three consecutive exchanges between sodium cyanoborohydride and deuterium oxide (D₂O) at a pH of 2. The final product crystallizes and is stored as a diethyl ether complex .
Industrial Production Methods: While specific industrial production methods are not extensively documented, the preparation generally follows the laboratory synthesis route, scaled up to meet industrial demands. The use of deuterium oxide and controlled reaction conditions ensures the high purity and yield of this compound .
Chemical Reactions Analysis
Types of Reactions: Sodium cyanoborodeuteride primarily undergoes reduction reactions. It is known for its ability to reduce imines and carbonyl compounds under mild conditions. This compound is less reactive than sodium borohydride, making it suitable for selective reductions .
Common Reagents and Conditions:
Reduction of Imines: this compound is used in the presence of an imine and a suitable solvent, such as methanol or ethanol. The reaction typically occurs at room temperature.
Reduction of Carbonyl Compounds: It can reduce aldehydes and ketones to their corresponding alcohols under mild acidic conditions
Major Products:
Reduction of Imines: Produces primary, secondary, or tertiary amines depending on the starting material.
Reduction of Carbonyl Compounds: Produces the corresponding alcohols
Scientific Research Applications
Sodium cyanoborodeuteride has a wide range of applications in scientific research:
Chemistry: Used in isotopic labeling and the synthesis of deuterated compounds.
Medicine: Utilized in the synthesis of deuterated drugs, which can have improved pharmacokinetic properties.
Mechanism of Action
Sodium cyanoborodeuteride exerts its effects through the transfer of deuterium to the substrate. The deuterium atoms replace hydrogen atoms in the target molecule, resulting in deuterated products. This process is facilitated by the mild reducing nature of the compound, which allows for selective reductions without over-reduction or unwanted side reactions .
Comparison with Similar Compounds
Sodium cyanoborohydride: The non-deuterated counterpart, which is more reactive and less selective in reductions.
Sodium borohydride: A stronger reducing agent used for more vigorous reductions.
Lithium aluminium hydride: A very strong reducing agent used for reductions that require more forceful conditions.
Uniqueness: Sodium cyanoborodeuteride is unique due to its deuterium content, which makes it particularly valuable for isotopic labeling and studies involving deuterium. Its mild reducing properties also make it suitable for selective reductions, where over-reduction needs to be avoided .
Properties
IUPAC Name |
sodium;cyano(trideuterio)boranuide | |
---|---|---|
Source | PubChem | |
URL | https://pubchem.ncbi.nlm.nih.gov | |
Description | Data deposited in or computed by PubChem | |
InChI |
InChI=1S/CH3BN.Na/c2-1-3;/h2H3;/q-1;+1/i2D3; | |
Source | PubChem | |
URL | https://pubchem.ncbi.nlm.nih.gov | |
Description | Data deposited in or computed by PubChem | |
InChI Key |
CVDUGUOQTVTBJH-MUTAZJQDSA-N | |
Source | PubChem | |
URL | https://pubchem.ncbi.nlm.nih.gov | |
Description | Data deposited in or computed by PubChem | |
Canonical SMILES |
[BH3-]C#N.[Na+] | |
Source | PubChem | |
URL | https://pubchem.ncbi.nlm.nih.gov | |
Description | Data deposited in or computed by PubChem | |
Isomeric SMILES |
[2H][B-]([2H])([2H])C#N.[Na+] | |
Source | PubChem | |
URL | https://pubchem.ncbi.nlm.nih.gov | |
Description | Data deposited in or computed by PubChem | |
Molecular Formula |
CH3BNNa | |
Source | PubChem | |
URL | https://pubchem.ncbi.nlm.nih.gov | |
Description | Data deposited in or computed by PubChem | |
DSSTOX Substance ID |
DTXSID50948802 | |
Record name | Sodium (cyanido-kappaC)(trihydrido)borate(1-) | |
Source | EPA DSSTox | |
URL | https://comptox.epa.gov/dashboard/DTXSID50948802 | |
Description | DSSTox provides a high quality public chemistry resource for supporting improved predictive toxicology. | |
Molecular Weight |
65.86 g/mol | |
Source | PubChem | |
URL | https://pubchem.ncbi.nlm.nih.gov | |
Description | Data deposited in or computed by PubChem | |
CAS No. |
25895-62-9 | |
Record name | Borate(1-), (cyano-κC)trihydro-d3-, sodium (1:1), (T-4)- | |
Source | CAS Common Chemistry | |
URL | https://commonchemistry.cas.org/detail?cas_rn=25895-62-9 | |
Description | CAS Common Chemistry is an open community resource for accessing chemical information. Nearly 500,000 chemical substances from CAS REGISTRY cover areas of community interest, including common and frequently regulated chemicals, and those relevant to high school and undergraduate chemistry classes. This chemical information, curated by our expert scientists, is provided in alignment with our mission as a division of the American Chemical Society. | |
Explanation | The data from CAS Common Chemistry is provided under a CC-BY-NC 4.0 license, unless otherwise stated. | |
Record name | Sodium (cyanido-kappaC)(trihydrido)borate(1-) | |
Source | EPA DSSTox | |
URL | https://comptox.epa.gov/dashboard/DTXSID50948802 | |
Description | DSSTox provides a high quality public chemistry resource for supporting improved predictive toxicology. | |
Record name | Sodium (cyano-C)[2H3]trihydroborate(1-) | |
Source | European Chemicals Agency (ECHA) | |
URL | https://echa.europa.eu/substance-information/-/substanceinfo/100.043.002 | |
Description | The European Chemicals Agency (ECHA) is an agency of the European Union which is the driving force among regulatory authorities in implementing the EU's groundbreaking chemicals legislation for the benefit of human health and the environment as well as for innovation and competitiveness. | |
Explanation | Use of the information, documents and data from the ECHA website is subject to the terms and conditions of this Legal Notice, and subject to other binding limitations provided for under applicable law, the information, documents and data made available on the ECHA website may be reproduced, distributed and/or used, totally or in part, for non-commercial purposes provided that ECHA is acknowledged as the source: "Source: European Chemicals Agency, http://echa.europa.eu/". Such acknowledgement must be included in each copy of the material. ECHA permits and encourages organisations and individuals to create links to the ECHA website under the following cumulative conditions: Links can only be made to webpages that provide a link to the Legal Notice page. | |
Retrosynthesis Analysis
AI-Powered Synthesis Planning: Our tool employs the Template_relevance Pistachio, Template_relevance Bkms_metabolic, Template_relevance Pistachio_ringbreaker, Template_relevance Reaxys, Template_relevance Reaxys_biocatalysis model, leveraging a vast database of chemical reactions to predict feasible synthetic routes.
One-Step Synthesis Focus: Specifically designed for one-step synthesis, it provides concise and direct routes for your target compounds, streamlining the synthesis process.
Accurate Predictions: Utilizing the extensive PISTACHIO, BKMS_METABOLIC, PISTACHIO_RINGBREAKER, REAXYS, REAXYS_BIOCATALYSIS database, our tool offers high-accuracy predictions, reflecting the latest in chemical research and data.
Strategy Settings
Precursor scoring | Relevance Heuristic |
---|---|
Min. plausibility | 0.01 |
Model | Template_relevance |
Template Set | Pistachio/Bkms_metabolic/Pistachio_ringbreaker/Reaxys/Reaxys_biocatalysis |
Top-N result to add to graph | 6 |
Feasible Synthetic Routes
Q1: What makes sodium cyanoborodeuteride advantageous for deuterium labeling?
A: this compound excels in selectively introducing deuterium atoms into molecules. This is evident in the synthesis of [2H5]-arecoline, where it proved more effective than sodium borodeuteride in incorporating deuterium into specific positions of the arecoline molecule []. This selectivity stems from the reagent's ability to participate in reductive amination reactions, replacing hydrogen with deuterium in the process.
Q2: Can you provide an example of this compound's stereochemical influence in a reaction?
A: Research shows that this compound can influence the stereochemical outcome of reactions. In the synthesis of specifically deuterated L-glutamic acid isomers, this compound reduction of precursor molecules resulted in a 75% inversion of configuration at the C-4 position []. This highlights the reagent's potential to control stereochemistry, a crucial aspect in synthesizing biologically active compounds with defined three-dimensional structures.
Q3: How is this compound used in structural studies of biomolecules?
A: Neutron diffraction studies benefit from the use of deuterium labeling, and this compound provides a way to achieve this. Researchers used this reagent to investigate glycation sites in diabetic tendon collagen []. By reacting collagen samples with this compound, specific sites within the collagen structure were labeled with deuterium. This isotopic labeling enabled the researchers to identify glycation hotspots in the collagen molecule using neutron diffraction techniques.
Q4: Are there any spectroscopic characterizations of this compound itself?
A: Yes, nuclear magnetic resonance (NMR) spectroscopy has been used to characterize this compound. Researchers have measured the ¹¹B and ²H spin-lattice relaxation times (T1) of this compound in solution []. These measurements provided valuable information about the molecular motions and interactions of the compound, further corroborated by computational methods.
Q5: Are there any computational studies related to this compound?
A: Yes, researchers have used computational methods to understand the properties of this compound. Specifically, ab initio molecular orbital theory was employed to calculate the electric field gradients in the cyanoborohydride ion, providing theoretical values for nuclear quadrupolar coupling constants []. These calculations offer a deeper understanding of the electronic structure and bonding characteristics of the compound, complementing experimental observations.
Disclaimer and Information on In-Vitro Research Products
Please be aware that all articles and product information presented on BenchChem are intended solely for informational purposes. The products available for purchase on BenchChem are specifically designed for in-vitro studies, which are conducted outside of living organisms. In-vitro studies, derived from the Latin term "in glass," involve experiments performed in controlled laboratory settings using cells or tissues. It is important to note that these products are not categorized as medicines or drugs, and they have not received approval from the FDA for the prevention, treatment, or cure of any medical condition, ailment, or disease. We must emphasize that any form of bodily introduction of these products into humans or animals is strictly prohibited by law. It is essential to adhere to these guidelines to ensure compliance with legal and ethical standards in research and experimentation.