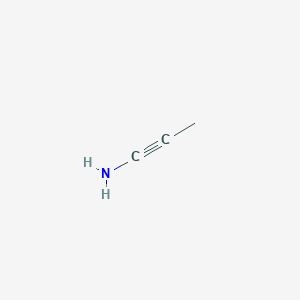
Propargylamine
Overview
Description
Propargylamine, also known as prop-2-yn-1-amine, is an organic compound with the chemical formula HC≡CCH₂NH₂. It is a colorless, odorless liquid that serves as a precursor to various other compounds. This compound is notable for its applications in organic synthesis and its role in the production of pharmaceuticals .
Mechanism of Action
Target of Action
Propargylamine primarily targets Monoamine Oxidase (MAO) , a flavin-containing enzyme that regulates the metabolic degradation of catecholamines and serotonin in the CNS and peripheral tissues . This compound derivatives such as pargyline, rasagiline, and selegiline are used against neurodegenerative disorders like Parkinson’s and Alzheimer’s diseases . Pargyline acts as an irreversible selective MAO-B inhibitor .
Mode of Action
This compound interacts with its targets, primarily MAO, by acting as an irreversible inhibitor . This means that once this compound binds to the MAO enzyme, it permanently deactivates it. This inhibition of MAO leads to an increase in the levels of monoamine neurotransmitters in the brain, which can help alleviate symptoms of certain neurodegenerative disorders .
Biochemical Pathways
The primary biochemical pathway affected by this compound is the monoamine oxidase pathway . By inhibiting MAO, this compound prevents the breakdown of monoamine neurotransmitters, leading to increased levels of these neurotransmitters in the brain . This can have downstream effects on various neurological processes, potentially alleviating symptoms of disorders like Parkinson’s and Alzheimer’s .
Result of Action
The molecular and cellular effects of this compound’s action primarily involve the prevention of monoamine neurotransmitter degradation . This can lead to increased levels of these neurotransmitters in the brain, potentially improving symptoms of neurodegenerative disorders. Additionally, this compound derivatives have been found to have neuroprotective effects, such as preventing apoptosis by reducing oxidative stress and stabilizing mitochondrial membranes .
Action Environment
The action, efficacy, and stability of this compound can be influenced by various environmental factors. For instance, the synthesis of this compound can be achieved via solvent-free synthetic approaches, which are considered more environmentally friendly . .
Biochemical Analysis
Biochemical Properties
Propargylamines play a major role in the synthesis of diverse biological activities and are used in treating neurogenetical disorders . They are known for their diverse biological activities and are produced by the KA2 coupling reaction, a well-explored and versatile method for forming C–C bonds in synthetic chemistry . This reaction is composed of ketone, amine, and alkyne .
Cellular Effects
Propargylamine derivatives such as selegiline and rasagiline have been found to have neuroprotective activities . These activities involve the prevention of the fall in mitochondrial membrane potential, attenuation of apoptotic cascades, inhibition of reactive oxygen species production, and up-regulation of neurotrophic factors and antioxidant activity .
Molecular Mechanism
The behavior of this compound is illustrated by its acylation benzoyl chloride to the amide . A Sonogashira coupling of the terminal alkyne end with another equivalent of benzoylchloride gives the dicarbonyl, a precursor to an oxazole .
Metabolic Pathways
It is known that propargylamines are produced by reactions of amines with propargyl halides , suggesting that they may be involved in amine metabolism.
Preparation Methods
Synthetic Routes and Reaction Conditions: Propargylamine can be synthesized through several methods, including the reaction of amines with propargyl halides. One common method involves the KA₂ coupling reaction, which includes ketone, amine, and alkyne components. This reaction is highly versatile and can be conducted under both thermal and non-conventional conditions .
Industrial Production Methods: In industrial settings, this compound is often produced using solvent-free methods to align with green chemistry principles. Copper(I) chloride catalyzed reactions and other metal-catalyzed processes are frequently employed to achieve high yields of this compound .
Chemical Reactions Analysis
Types of Reactions: Propargylamine undergoes various chemical reactions, including:
Oxidation: this compound can be oxidized to form aldehydes and ketones.
Reduction: It can be reduced to form primary amines.
Substitution: this compound can participate in nucleophilic substitution reactions.
Common Reagents and Conditions:
Oxidation: Common oxidizing agents include potassium permanganate and chromium trioxide.
Reduction: Reducing agents such as lithium aluminum hydride are used.
Substitution: Reagents like benzoyl chloride are employed in substitution reactions.
Major Products: The major products formed from these reactions include various amides, aldehydes, and ketones, depending on the specific reaction conditions and reagents used .
Scientific Research Applications
Propargylamine has a wide range of applications in scientific research:
Chemistry: It is used as a building block in the synthesis of heterocyclic compounds and other complex molecules.
Biology: this compound derivatives are studied for their potential neuroprotective effects.
Industry: this compound is used in the production of various pharmaceuticals and agrochemicals.
Comparison with Similar Compounds
Rasagiline: An irreversible inhibitor of monoamine oxidase used in the treatment of Parkinson’s disease.
Selegiline: Another monoamine oxidase inhibitor with neuroprotective properties.
Uniqueness: Propargylamine is unique due to its triple bond and nitrogen bond, which confer high reactivity and versatility in organic synthesis. This makes it a valuable precursor for the synthesis of various N-heterocycles and other functional groups .
Properties
IUPAC Name |
prop-2-yn-1-amine | |
---|---|---|
Source | PubChem | |
URL | https://pubchem.ncbi.nlm.nih.gov | |
Description | Data deposited in or computed by PubChem | |
InChI |
InChI=1S/C3H5N/c1-2-3-4/h1H,3-4H2 | |
Source | PubChem | |
URL | https://pubchem.ncbi.nlm.nih.gov | |
Description | Data deposited in or computed by PubChem | |
InChI Key |
JKANAVGODYYCQF-UHFFFAOYSA-N | |
Source | PubChem | |
URL | https://pubchem.ncbi.nlm.nih.gov | |
Description | Data deposited in or computed by PubChem | |
Canonical SMILES |
C#CCN | |
Source | PubChem | |
URL | https://pubchem.ncbi.nlm.nih.gov | |
Description | Data deposited in or computed by PubChem | |
Molecular Formula |
C3H5N | |
Source | PubChem | |
URL | https://pubchem.ncbi.nlm.nih.gov | |
Description | Data deposited in or computed by PubChem | |
DSSTOX Substance ID |
DTXSID30862951 | |
Record name | 2-Propyn-1-amine | |
Source | EPA DSSTox | |
URL | https://comptox.epa.gov/dashboard/DTXSID30862951 | |
Description | DSSTox provides a high quality public chemistry resource for supporting improved predictive toxicology. | |
Molecular Weight |
55.08 g/mol | |
Source | PubChem | |
URL | https://pubchem.ncbi.nlm.nih.gov | |
Description | Data deposited in or computed by PubChem | |
CAS No. |
2450-71-7 | |
Record name | Propargylamine | |
Source | CAS Common Chemistry | |
URL | https://commonchemistry.cas.org/detail?cas_rn=2450-71-7 | |
Description | CAS Common Chemistry is an open community resource for accessing chemical information. Nearly 500,000 chemical substances from CAS REGISTRY cover areas of community interest, including common and frequently regulated chemicals, and those relevant to high school and undergraduate chemistry classes. This chemical information, curated by our expert scientists, is provided in alignment with our mission as a division of the American Chemical Society. | |
Explanation | The data from CAS Common Chemistry is provided under a CC-BY-NC 4.0 license, unless otherwise stated. | |
Record name | Propargylamine | |
Source | DTP/NCI | |
URL | https://dtp.cancer.gov/dtpstandard/servlet/dwindex?searchtype=NSC&outputformat=html&searchlist=80642 | |
Description | The NCI Development Therapeutics Program (DTP) provides services and resources to the academic and private-sector research communities worldwide to facilitate the discovery and development of new cancer therapeutic agents. | |
Explanation | Unless otherwise indicated, all text within NCI products is free of copyright and may be reused without our permission. Credit the National Cancer Institute as the source. | |
Record name | 2-Propyn-1-amine | |
Source | EPA Chemicals under the TSCA | |
URL | https://www.epa.gov/chemicals-under-tsca | |
Description | EPA Chemicals under the Toxic Substances Control Act (TSCA) collection contains information on chemicals and their regulations under TSCA, including non-confidential content from the TSCA Chemical Substance Inventory and Chemical Data Reporting. | |
Record name | 2-Propyn-1-amine | |
Source | EPA DSSTox | |
URL | https://comptox.epa.gov/dashboard/DTXSID30862951 | |
Description | DSSTox provides a high quality public chemistry resource for supporting improved predictive toxicology. | |
Record name | Prop-2-ynylamine | |
Source | European Chemicals Agency (ECHA) | |
URL | https://echa.europa.eu/substance-information/-/substanceinfo/100.017.740 | |
Description | The European Chemicals Agency (ECHA) is an agency of the European Union which is the driving force among regulatory authorities in implementing the EU's groundbreaking chemicals legislation for the benefit of human health and the environment as well as for innovation and competitiveness. | |
Explanation | Use of the information, documents and data from the ECHA website is subject to the terms and conditions of this Legal Notice, and subject to other binding limitations provided for under applicable law, the information, documents and data made available on the ECHA website may be reproduced, distributed and/or used, totally or in part, for non-commercial purposes provided that ECHA is acknowledged as the source: "Source: European Chemicals Agency, http://echa.europa.eu/". Such acknowledgement must be included in each copy of the material. ECHA permits and encourages organisations and individuals to create links to the ECHA website under the following cumulative conditions: Links can only be made to webpages that provide a link to the Legal Notice page. | |
Synthesis routes and methods I
Procedure details
Synthesis routes and methods II
Procedure details
Synthesis routes and methods III
Procedure details
Synthesis routes and methods IV
Procedure details
Synthesis routes and methods V
Procedure details
Retrosynthesis Analysis
AI-Powered Synthesis Planning: Our tool employs the Template_relevance Pistachio, Template_relevance Bkms_metabolic, Template_relevance Pistachio_ringbreaker, Template_relevance Reaxys, Template_relevance Reaxys_biocatalysis model, leveraging a vast database of chemical reactions to predict feasible synthetic routes.
One-Step Synthesis Focus: Specifically designed for one-step synthesis, it provides concise and direct routes for your target compounds, streamlining the synthesis process.
Accurate Predictions: Utilizing the extensive PISTACHIO, BKMS_METABOLIC, PISTACHIO_RINGBREAKER, REAXYS, REAXYS_BIOCATALYSIS database, our tool offers high-accuracy predictions, reflecting the latest in chemical research and data.
Strategy Settings
Precursor scoring | Relevance Heuristic |
---|---|
Min. plausibility | 0.01 |
Model | Template_relevance |
Template Set | Pistachio/Bkms_metabolic/Pistachio_ringbreaker/Reaxys/Reaxys_biocatalysis |
Top-N result to add to graph | 6 |
Feasible Synthetic Routes
Disclaimer and Information on In-Vitro Research Products
Please be aware that all articles and product information presented on BenchChem are intended solely for informational purposes. The products available for purchase on BenchChem are specifically designed for in-vitro studies, which are conducted outside of living organisms. In-vitro studies, derived from the Latin term "in glass," involve experiments performed in controlled laboratory settings using cells or tissues. It is important to note that these products are not categorized as medicines or drugs, and they have not received approval from the FDA for the prevention, treatment, or cure of any medical condition, ailment, or disease. We must emphasize that any form of bodily introduction of these products into humans or animals is strictly prohibited by law. It is essential to adhere to these guidelines to ensure compliance with legal and ethical standards in research and experimentation.