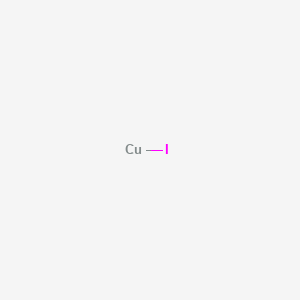
Iodocopper
- Click on QUICK INQUIRY to receive a quote from our team of experts.
- With the quality product at a COMPETITIVE price, you can focus more on your research.
Overview
Description
Iodocopper (CuI), also known as copper(I) iodide, is an inorganic compound with the chemical formula CuI. It is a white to grayish solid with a density of 5.62 g/mL and a melting point of 605°C . Its molecular structure consists of a tetrahedral coordination geometry, forming a three-dimensional network stabilized by iodide ions. CuI is sparingly soluble in water but dissolves in solutions containing iodide ions (e.g., KI) or coordinating solvents like acetonitrile.
Preparation Methods
Synthetic Routes and Reaction Conditions: Iodocopper can be synthesized through several methods:
Reaction of Copper and Iodine: Heating copper and iodine in concentrated hydroiodic acid produces this compound.
In the laboratory, this compound is often prepared by mixing an aqueous solution of potassium iodide with a soluble copper(II) salt such as copper(II) sulfate. The reaction proceeds as follows:Reaction of Copper(II) Sulfate and Potassium Iodide: 2Cu2++4I−→2CuI+I2
Biological Activity
Iodocopper, specifically in the form of copper iodide (CuI), has garnered significant attention in recent years due to its diverse biological activities. This article delves into the biological mechanisms of this compound, focusing on its antimicrobial properties, cellular interactions, and potential therapeutic applications.
1. Antimicrobial Properties
Copper Iodide Nanoparticles
Research has demonstrated that copper iodide nanoparticles exhibit considerable antibacterial activity. A study highlighted the effectiveness of CuI nanoparticles against various bacterial strains, suggesting their potential as a new class of antimicrobial agents. The mechanism of action involves the generation of reactive oxygen species (ROS) and the disruption of bacterial cell membranes, leading to cell death .
Table 1: Antimicrobial Efficacy of CuI Nanoparticles
Bacterial Strain | Minimum Inhibitory Concentration (MIC) | Mechanism of Action |
---|---|---|
Escherichia coli | 32 µg/mL | Membrane disruption, ROS generation |
Staphylococcus aureus | 16 µg/mL | Membrane disruption, oxidative stress |
Pseudomonas aeruginosa | 64 µg/mL | Membrane disruption, interference with metabolism |
2. Cellular Interactions
Cellular Uptake and Toxicity
The biological activity of this compound is also linked to its interaction with cellular components. A study involving HepG2 liver cancer cells showed that biodegradable Cu-I clusters could induce cell death through mitochondrial dysfunction. The release of free copper and iodine ions was observed to significantly impair cellular ATP production, indicating a shift in cellular metabolism towards apoptosis .
Table 2: Effects of Cu-I on HepG2 Cells
Treatment Condition | ATP Production (%) | Cell Viability (%) | Mitochondrial Membrane Potential (ΔΨm) |
---|---|---|---|
Control | 100 | 100 | Normal |
Cu-I (1.25 µg/mL) | 41.4 | 70 | Depolarized |
Cu-I + X-ray Irradiation | 8.1 | 30 | Severely Depolarized |
3. Case Studies
Case Study: Antiviral Activity
A notable case study explored the antiviral properties of nanosized CuI particles against the H1N1 influenza virus. The results indicated that these particles could effectively inactivate the virus, providing a promising avenue for developing antiviral therapies . The study emphasized that the mechanism involved direct interaction with viral particles, leading to structural damage.
Case Study: Green Synthesis and Application
Another case study focused on the green synthesis of copper iodide nanoparticles using natural extracts from Hibiscus rosa-sinensis L. This approach not only enhanced the antimicrobial efficacy but also reduced environmental impact compared to conventional synthesis methods . The coated cotton fabric exhibited significant antibacterial activity against pathogens like E. coli and S. aureus.
4. Conclusion
This compound, particularly in the form of copper iodide nanoparticles, shows promising biological activity through its antimicrobial and cytotoxic effects. Its ability to induce oxidative stress in microbial cells and disrupt mitochondrial function in human cells highlights its dual potential as an antimicrobial agent and a therapeutic compound in cancer treatment. Ongoing research is essential to fully understand its mechanisms and optimize its applications in medicine.
Scientific Research Applications
Organic Synthesis and Catalysis
Cross-Coupling Reactions
CuI is a pivotal catalyst in several cross-coupling reactions, which are essential for forming carbon-carbon bonds in organic synthesis. Notable reactions include:
- Ullmann Coupling : CuI facilitates the formation of C–N, C–O, and C–S bonds under mild conditions, overcoming limitations of traditional methods that often require harsh environments .
- Sonogashira Coupling : This reaction enables the coupling of terminal alkynes with aryl or vinyl halides, producing conjugated enynes valuable in pharmaceuticals and materials science. CuI enhances reaction efficiency by stabilizing intermediates .
Oxidation Reactions
CuI serves as a co-catalyst in various oxidation processes:
- Oxidative Cyclization : It promotes the formation of cyclic compounds from linear precursors, crucial for synthesizing heterocycles and complex natural products .
- Oxidative Coupling : CuI can couple identical organic molecules to form dimers or oligomers, aiding in the synthesis of polymeric materials .
Semiconductor Applications
CuI is recognized as a promising p-type semiconductor due to its adjustable conductivity and transparency. Its applications include:
- Transparent Conductors : CuI can be used to create transparent electrodes for displays and solar cells, thanks to its high transparency in the visible spectrum and good electrical properties .
- Transistors and Diodes : The material has been developed into thin films for use in p-channel transistors and heterojunction diodes, showcasing excellent hole mobility and conductivity .
Application | Description |
---|---|
Transparent Electrodes | Used in photovoltaic devices and displays due to high transparency. |
P-Channel Transistors | Exhibits high hole mobility (up to 44 cm²/V·s) for efficient charge transport. |
Heterojunction Diodes | Forms efficient junctions with n-type materials for electronic devices. |
Antiviral Applications
Recent studies have highlighted CuI's effectiveness against viruses, particularly SARS-CoV-2:
- Virucidal Activity : CuI nanoparticles demonstrated rapid inactivation of SARS-CoV-2 when incorporated into films and fabrics. The mechanism involves the generation of reactive oxygen species that damage viral proteins and genetic material .
Material Type | Viral Inactivation Rate | Mechanism of Action |
---|---|---|
CuI Nanoparticle Dispersion | High | Induces destruction of viral proteins via reactive oxygen species. |
CuI-Doped Films | Rapid | Effective against high viral titers on surfaces. |
Materials Science
CuI's unique properties allow it to be integrated into advanced materials:
- Optical Sensors and Light Emitting Diodes (LEDs) : CuI-based materials exhibit tunable photophysical properties suitable for optoelectronic applications .
- Polymerization Reactions : It is used to mediate radical polymerizations, leading to the development of new copolymers with tailored properties .
Q & A
Basic Research Questions
Q. What are the optimal synthetic routes for preparing high-purity Iodocopper, and how can purity be validated?
Methodological Answer: High-purity this compound (CuI) can be synthesized via solvothermal methods using copper(II) precursors and iodide sources in polar solvents (e.g., acetonitrile or dimethylformamide). Purification involves recrystallization or vacuum sublimation. Validate purity using:
- X-ray Diffraction (XRD): Confirm crystalline structure matches reference data (JCPDS).
- Elemental Analysis: Verify stoichiometric Cu:I ratios (e.g., ±0.5% deviation).
- Thermogravimetric Analysis (TGA): Assess thermal stability and residual solvent content. Reproducibility requires meticulous documentation of reaction conditions (temperature, solvent ratios, stirring rates) as per experimental guidelines .
Q. Which spectroscopic techniques are most effective for characterizing this compound’s structural and electronic properties?
Methodological Answer: A combination of techniques ensures comprehensive characterization:
Q. How should researchers design experiments to study this compound’s reactivity in cross-coupling reactions?
Methodological Answer: Use controlled variables:
- Catalyst Loading: Test 1-10 mol% to identify optimal efficiency.
- Solvent Effects: Compare polar aprotic (DMF, DMSO) vs. non-polar solvents (toluene).
- Temperature Gradients: Conduct reactions at 25°C, 50°C, and 80°C to assess kinetic vs. thermodynamic control. Document all parameters systematically to enable replication, aligning with guidelines for experimental rigor .
Advanced Research Questions
Q. How can computational methods like DFT resolve contradictions in this compound’s reaction mechanisms reported across studies?
Methodological Answer: Density Functional Theory (DFT) simulations can model transition states and intermediates to reconcile conflicting mechanistic proposals. Key steps:
- Geometry Optimization: Use B3LYP/6-311G** basis sets for Cu and I.
- Energy Profiling: Compare activation barriers for proposed pathways (e.g., oxidative addition vs. π-coordination).
- Solvent Modeling: Apply implicit solvation models (e.g., PCM) to account for solvent effects. Validate computational results with kinetic isotope effects (KIEs) or in-situ spectroscopic data .
Q. What strategies address discrepancies in this compound’s catalytic efficiency across heterogeneous vs. homogeneous systems?
Methodological Answer: Conduct a systematic review to identify variables causing discrepancies:
- Catalyst Dispersion: Compare colloidal vs. molecularly dispersed CuI.
- Support Matrix Effects: Test this compound on SiO₂, Al₂O₃, or carbon supports.
- Leaching Tests: Use hot filtration or three-phase tests to confirm heterogeneity. Apply meta-analysis frameworks (e.g., PRISMA) to synthesize findings from ≥20 studies, ensuring statistical power .
Q. How can researchers design studies to probe this compound’s role in photophysical applications while mitigating experimental bias?
Methodological Answer: Implement blinding and randomization:
- Blinded Sample Preparation: Assign codes to CuI samples to prevent operator bias.
- Control Groups: Include non-irradiated samples and CuCl/CuBr analogs.
- Repeated Trials: Conduct ≥3 independent experiments under identical conditions. Use multivariate analysis (ANOVA) to distinguish significant photoluminescence quantum yield (PLQY) variations .
Q. Data Contradiction Analysis
Q. What methodologies reconcile conflicting reports on this compound’s stability under ambient conditions?
Methodological Answer: Address contradictions via:
- Environmental Controls: Repeat experiments in gloveboxes (O₂ < 0.1 ppm, H₂O < 1 ppm).
- Surface Analysis: Use XPS or Auger spectroscopy to detect oxide/hydroxide layers.
- Long-Term Stability Studies: Monitor XRD/FT-IR weekly for 6 months. Cross-reference findings with historical data from documentary analysis .
Comparison with Similar Compounds
Comparison with Similar Copper(I) Halides
CuI is part of the copper(I) halide family, which includes CuCl (copper(I) chloride) and CuBr (copper(I) bromide). Below is a detailed comparison:
Physical and Chemical Properties
Property | CuI | CuCl | CuBr |
---|---|---|---|
Density (g/cm³) | 5.62 | 4.14 | 4.71 |
Melting Point (°C) | 605 | 423 | 492 |
Solubility in Water | 0.001 g/100 mL | 0.06 g/100 mL | 0.05 g/100 mL |
Coordination Geometry | Tetrahedral | Tetrahedral | Tetrahedral |
Key Observations :
- CuI has the highest density and melting point among copper(I) halides due to stronger van der Waals interactions from larger iodide ions .
- CuCl and CuBr exhibit marginally higher water solubility, attributed to smaller ionic radii and lower lattice energy .
Reactivity and Catalytic Efficiency
(a) Catalytic Activity in Cross-Coupling Reactions
- CuI outperforms CuCl and CuBr in Sonogashira coupling, achieving yields >90% under mild conditions (e.g., 110°C in DMSO) .
- CuCl is preferred in Sandmeyer reactions due to its higher solubility in aqueous HCl, facilitating aryl diazonium salt transformations .
(b) Ligand Coordination
- CuI forms stable complexes with soft ligands like thiourea (logP = 2.03) and phosphites (PSA = 41.28), while CuCl favors hard ligands such as amines .
- Example: Tris(N,N’-diethylthiourea-S)iodocopper(I) exhibits superior antioxidant activity compared to analogous CuCl complexes, likely due to enhanced iodide-mediated redox stability .
Key Findings :
- CuI derivatives show lower antitumor activity compared to parent compounds like gambogic acid (GA), likely due to steric hindrance from iodide .
- CuCl complexes exhibit stronger antibacterial effects, possibly due to chloride’s higher electronegativity and membrane permeability .
Stability and Industrial Handling
Properties
CAS No. |
7681-65-4 |
---|---|
Molecular Formula |
CuI |
Molecular Weight |
190.45 g/mol |
IUPAC Name |
copper(1+);iodide |
InChI |
InChI=1S/Cu.HI/h;1H/q+1;/p-1 |
InChI Key |
LSXDOTMGLUJQCM-UHFFFAOYSA-M |
SMILES |
[Cu]I |
Canonical SMILES |
[Cu+].[I-] |
boiling_point |
About 1290 °C |
Color/Form |
DENSE POWDER OR CUBIC CRYSTALS (ZINC BLENDE STRUCTURE) White cubic crystals White to brownish yellow powde |
density |
5.67 |
melting_point |
606 °C |
Key on ui other cas no. |
7681-65-4 |
physical_description |
White to brownish-yellow solid; [Hawley] Insoluble in water; [Ullmann] White to light brown powder chunks with a weak odor; [Alfa Aesar MSDS] |
shelf_life |
It is generally stable in air & is less photosensitive than the chloride or bromide. |
solubility |
Insoluble in dilute acid solutions Dissolves in ammonia solution, alkali iodide and cyanide solutions, and dilute hydrochloric acid Soluble in ammonia and potassium iodide solutions; insoluble in water 0.008 g/100 cc water @ 18 °C |
Synonyms |
Copper Monoiodide; Copper(1+) Iodide; Copper(I) Iodide; Cuprous Iodide; Cuprous Iodide (CuI); |
vapor_pressure |
10 MM HG @ 656 °C |
Origin of Product |
United States |
Disclaimer and Information on In-Vitro Research Products
Please be aware that all articles and product information presented on BenchChem are intended solely for informational purposes. The products available for purchase on BenchChem are specifically designed for in-vitro studies, which are conducted outside of living organisms. In-vitro studies, derived from the Latin term "in glass," involve experiments performed in controlled laboratory settings using cells or tissues. It is important to note that these products are not categorized as medicines or drugs, and they have not received approval from the FDA for the prevention, treatment, or cure of any medical condition, ailment, or disease. We must emphasize that any form of bodily introduction of these products into humans or animals is strictly prohibited by law. It is essential to adhere to these guidelines to ensure compliance with legal and ethical standards in research and experimentation.