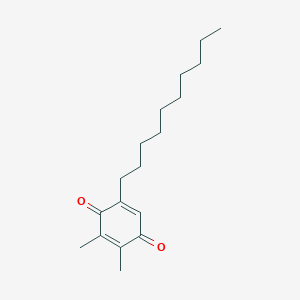
Decylplastoquinone
Overview
Description
Decylplastoquinone (DPQ, CAS 112055-76-2) is a synthetic analog of endogenous plastoquinones, featuring a decyl side chain instead of the natural prenyl tail. It plays a critical role in photosynthesis research as a redox-active molecule, mimicking the function of plastoquinone-9 (PQ-9) in electron transport chains. DPQ is widely utilized in experimental systems to study photosystem II (PSII) kinetics, cytochrome b6f activity, and as an internal standard in analytical chemistry . Its synthetic nature allows for controlled applications in reconstituted enzymatic assays, where solubility and stability are paramount .
Preparation Methods
Synthetic Routes and Reaction Conditions: Decylplastoquinone can be synthesized through a multi-step process involving the alkylation of 2,3-dimethyl-1,4-benzoquinone with decyl bromide under basic conditions . The reaction typically requires a strong base such as potassium carbonate and an aprotic solvent like dimethylformamide. The reaction mixture is heated to facilitate the alkylation process, followed by purification through column chromatography.
Industrial Production Methods: In an industrial setting, the production of this compound may involve similar synthetic routes but on a larger scale. The use of continuous flow reactors can enhance the efficiency and yield of the reaction. Additionally, advanced purification techniques such as high-performance liquid chromatography (HPLC) are employed to ensure the purity of the final product .
Types of Reactions:
Common Reagents and Conditions:
Oxidation: Potassium permanganate, hydrogen peroxide.
Reduction: Sodium borohydride, lithium aluminum hydride.
Substitution: Alkyl or aryl halides, strong bases.
Major Products:
Oxidation: Quinone derivatives.
Reduction: Hydroquinone derivatives.
Substitution: Various substituted benzoquinones.
Scientific Research Applications
Decylplastoquinone has garnered significant attention in various scientific fields due to its redox-active properties :
Chemistry: Used as a model compound for studying electron transfer processes and redox reactions.
Biology: Investigated for its role in photosynthetic electron transport chains and as a potential antioxidant.
Medicine: Explored for its potential therapeutic applications in preventing oxidative stress-related diseases.
Industry: Utilized in the synthesis of advanced materials and as a component in redox-active polymers.
Mechanism of Action
Decylplastoquinone exerts its effects primarily through its role in electron transfer processes . It acts as an electron carrier in biological systems, facilitating the transfer of electrons between different molecules. This process is crucial in maintaining the redox balance within cells and preventing oxidative damage. The compound targets various molecular pathways involved in electron transport, particularly within the mitochondria .
Comparison with Similar Compounds
Structural and Functional Differences
Decylplastoquinone (DPQ) vs. Plastoquinone-9 (PQ-9)
- Structure: DPQ: Short decyl chain (C10, saturated hydrocarbon). PQ-9: Long isoprenoid tail (nine isoprene units, ~C45).
- Function: Both act as mobile electron carriers between PSII and cytochrome b6f. In reconstituted systems, DPQ restores DPC→DCIP photoreduction activity in PSII complexes, though with lower efficiency than PQ-9 due to structural constraints .
DPQ vs. 3-(3,4-Dichlorophenyl)-1,1-Dimethylurea (DCMU)
- Mechanism :
- Applications :
Redox Properties and Enzymatic Activity
- Cytochrome b6f Assays: DPQ is dissolved in ethanol for redox activity measurements, demonstrating a differential extinction coefficient (Δε₅₅₄–₅₄₃ ≈ 25 mM⁻¹ cm⁻¹) similar to natural quinones.
- Methane Monooxygenase (pMMO) Studies: DPQ is used alongside PSII components to mediate electron transfer in methane oxidation assays, highlighting its versatility as a synthetic redox mediator .
Research Implications and Limitations
DPQ’s synthetic design bridges the gap between natural quinones and experimental feasibility. While it cannot fully replicate PQ-9’s membrane dynamics, its stability and solubility make it indispensable in in vitro studies. Future work should explore hybrid analogs with tunable side chains to better mimic natural systems .
Biological Activity
Decylplastoquinone (DPQ) is a synthetic analogue of plastoquinone, a crucial component in the electron transport chain of photosynthesis and respiration. Its biological activity is primarily linked to its role in redox reactions and interactions with various proteins, particularly in plant systems. This article delves into the molecular mechanisms, structural insights, and biological implications of DPQ, supported by relevant research findings.
This compound functions as an electron carrier in the thylakoid membranes of chloroplasts. Its interaction with the cytochrome b6f complex is particularly significant. Recent studies utilizing high-resolution cryo-electron microscopy have revealed that DPQ occupies specific sites within the cytochrome b6f complex, facilitating electron transfer during photosynthesis.
- Binding Sites : DPQ adopts a unique position in the Qn site of the cytochrome b6f complex, distinct from natural substrates. This positioning is critical for its role in catalysis and electron transfer processes .
- Conformational Dynamics : The dynamic movement of the iron-sulfur protein (ISP) within the cytochrome b6f complex is influenced by DPQ binding. Structural studies indicate that the ISP undergoes significant conformational changes upon interaction with DPQ, which may enhance substrate exchange mechanisms .
Biological Implications
The biological activity of DPQ extends beyond its role as an electron carrier. It has been shown to influence various biochemical pathways and physiological responses in plants.
- Inhibition of Kinase Activity : Research indicates that reduced forms of DPQ can inhibit the autokinase activity of certain chloroplast proteins, such as CSK (chloroplast sensor kinase). This inhibition is linked to redox state changes within iron-sulfur clusters, suggesting a regulatory role for DPQ in chloroplast signaling pathways .
- Photosynthetic Efficiency : The presence of DPQ affects the efficiency of photosynthetic processes by modulating electron flow through the photosystem II (PSII) complex. Studies show that DPQ can alter fluorescence induction profiles, indicating changes in electron transport dynamics .
Case Studies and Research Findings
Several studies have highlighted the biological activity and significance of this compound:
- Photosynthetic Performance : A study on Arabidopsis demonstrated that varying concentrations of DPQ influenced photosynthetic rates and chlorophyll fluorescence parameters, indicating its role in optimizing light energy capture .
- Redox Regulation : In a controlled experiment, treatment with DPQ led to significant alterations in redox states within chloroplasts, impacting downstream signaling pathways associated with stress responses .
- Structural Analysis : High-resolution structural analyses have provided insights into how DPQ interacts with key proteins involved in photosynthesis. The observed structural conformations reveal potential pathways for improving plant resilience through targeted manipulation of plastoquinone analogues .
Data Tables
The following table summarizes key findings related to the biological activity of this compound:
Q & A
Q. Basic: What is the role of decylplastoquinone in membrane protein studies?
Answer:
this compound (dPQ) is a synthetic analog of plastoquinone, primarily used to study electron transport mechanisms in photosynthetic systems. In membrane protein research, it facilitates assays involving cytochrome b6f complexes, where it acts as a redox mediator. For example, dPQ is dissolved in ethanol (25 mM concentration) and integrated into activity assays to measure electron transfer rates under controlled conditions . Its lipophilic properties enable interactions with integral membrane proteins, making it critical for mimicking native quinone behavior in vitro.
Key Applications:
- Redox activity measurements in chloroplasts.
- Stabilizing detergent-lipid-protein interactions during membrane protein extraction .
Q. Basic: How is this compound synthesized and characterized for experimental use?
Answer:
Synthesis involves alkylation of plastoquinone precursors followed by purification via high-performance liquid chromatography (HPLC). Characterization requires:
Nuclear Magnetic Resonance (NMR): To confirm alkyl chain length and quinone headgroup structure.
Mass Spectrometry (MS): For molecular weight validation.
UV-Vis Spectroscopy: To verify redox-active absorbance peaks (e.g., ~254 nm for oxidized dPQ).
Experimental protocols must detail solvent compatibility (e.g., ethanol for solubility) and storage conditions (-20°C to prevent degradation) .
Q. Advanced: What methodological challenges arise in measuring dPQ’s activity in vitro?
Answer:
Challenges include:
- Solvent Interference: Ethanol, used to dissolve dPQ, may denature proteins at high concentrations. Mitigation involves optimizing solvent-to-buffer ratios .
- Redox State Stability: dPQ’s redox activity is sensitive to oxygen; assays require anaerobic conditions or inert gas purging (e.g., hydrogen or nitrogen) .
- Signal-to-Noise Ratio: Spectrophotometric measurements (e.g., Cary 4000) must account for background absorbance from detergents like UDM-Sol .
Table 1: Optimized Conditions for dPQ Activity Assays
Parameter | Optimal Range | Reference |
---|---|---|
dPQ Concentration | 25 mM in ethanol | |
Assay Temperature | 25°C ± 0.5°C | |
Buffer pH | 7.4–7.8 (Tris-HCl) |
Q. Advanced: How can researchers resolve contradictions in dPQ interaction data across studies?
Answer:
Contradictions often stem from:
- Variability in Detergent Use: Different detergents (e.g., UDM-Sol vs. DDM) alter dPQ’s accessibility to proteins. Standardize detergent concentrations and critical micelle concentrations (CMCs) .
- Assay Heterogeneity: Differences in redox potential measurements due to buffer ionic strength or temperature fluctuations. Use calibrated spectrophotometers with thermostat controls .
- Data Normalization: Normalize activity rates to protein concentration (e.g., μmol·min⁻¹·mg⁻¹) and include negative controls (e.g., ethanol-only) .
Strategies:
- Replicate experiments across ≥3 independent preparations.
- Apply statistical frameworks (e.g., ANOVA with post-hoc tests) to identify outliers .
Q. Methodological: What analytical techniques are optimal for quantifying dPQ in complex mixtures?
Answer:
- Reverse-Phase HPLC: Separates dPQ from lipids/proteins using C18 columns and isocratic elution (acetonitrile:water = 85:15).
- Electrochemical Detection: Measures redox currents at specific potentials (e.g., +0.6 V vs. Ag/AgCl) .
- LC-MS/MS: Provides high sensitivity for trace quantification (LOQ ≈ 10 nM) but requires deuterated internal standards .
Validation:
- Spike-and-recovery tests in biological matrices (e.g., chloroplast extracts).
- Cross-validate with UV-Vis spectra to confirm purity .
Q. Experimental Design: How to study dPQ’s effects on electron transport chains?
Answer:
Define PICOT Framework:
- Population: Isolated cytochrome b6f complexes.
- Intervention: dPQ concentration gradients (0–50 μM).
- Comparison: Native plastoquinone-9.
- Outcome: Electron transfer rate (ΔA₅₅₀/min).
- Time: 0–60 min .
Controls: Include detergent-only and quinone-free buffers.
Data Collection: Use stopped-flow spectrophotometry for kinetic analysis .
Q. Advanced: How to address variability in dPQ assay results?
Answer:
- Source Tracing: Document reagent lot numbers (e.g., Sigma-Aldrich dPQ vs. alternative suppliers).
- Environmental Controls: Monitor lab temperature/humidity, which affect ethanol evaporation rates.
- Statistical Modeling: Apply mixed-effects models to account for batch-to-batch variability .
Q. Advanced: What are the implications of dPQ’s solubility in different solvents?
Answer:
dPQ’s solubility in ethanol (25 mM) vs. water (<0.1 mM) impacts:
- Membrane Incorporation Efficiency: Ethanol enhances lipid bilayer penetration but requires <1% (v/v) to avoid protein denaturation.
- Assay Reproducibility: Pre-dissolve dPQ in ethanol and dilute into aqueous buffers immediately before use .
Q. Methodological: How to ensure reproducibility in dPQ-based experiments?
Answer:
- Detailed Protocols: Specify centrifugation speeds (e.g., 100,000 × g for membrane pellets), filter pore sizes (0.22 μm), and degassing steps for buffers .
- Open Data: Share raw spectrophotometric traces and HPLC chromatograms as supplementary materials .
Research Gaps: What unanswered questions exist about dPQ’s mechanism?
Answer:
- Long-Term Stability: How do repeated freeze-thaw cycles affect dPQ’s redox capacity?
- Cross-Species Compatibility: Does dPQ function identically in cyanobacterial vs. plant cytochrome systems?
- Alternative Applications: Can dPQ modulate non-photosynthetic membrane proteins (e.g., mitochondrial Complex III)? .
Properties
IUPAC Name |
5-decyl-2,3-dimethylcyclohexa-2,5-diene-1,4-dione | |
---|---|---|
Source | PubChem | |
URL | https://pubchem.ncbi.nlm.nih.gov | |
Description | Data deposited in or computed by PubChem | |
InChI |
InChI=1S/C18H28O2/c1-4-5-6-7-8-9-10-11-12-16-13-17(19)14(2)15(3)18(16)20/h13H,4-12H2,1-3H3 | |
Source | PubChem | |
URL | https://pubchem.ncbi.nlm.nih.gov | |
Description | Data deposited in or computed by PubChem | |
InChI Key |
APNQQQRDLMWNLM-UHFFFAOYSA-N | |
Source | PubChem | |
URL | https://pubchem.ncbi.nlm.nih.gov | |
Description | Data deposited in or computed by PubChem | |
Canonical SMILES |
CCCCCCCCCCC1=CC(=O)C(=C(C1=O)C)C | |
Source | PubChem | |
URL | https://pubchem.ncbi.nlm.nih.gov | |
Description | Data deposited in or computed by PubChem | |
Molecular Formula |
C18H28O2 | |
Source | PubChem | |
URL | https://pubchem.ncbi.nlm.nih.gov | |
Description | Data deposited in or computed by PubChem | |
DSSTOX Substance ID |
DTXSID60409010 | |
Record name | Decylplastoquinone | |
Source | EPA DSSTox | |
URL | https://comptox.epa.gov/dashboard/DTXSID60409010 | |
Description | DSSTox provides a high quality public chemistry resource for supporting improved predictive toxicology. | |
Molecular Weight |
276.4 g/mol | |
Source | PubChem | |
URL | https://pubchem.ncbi.nlm.nih.gov | |
Description | Data deposited in or computed by PubChem | |
CAS No. |
112055-76-2 | |
Record name | Decylplastoquinone | |
Source | CAS Common Chemistry | |
URL | https://commonchemistry.cas.org/detail?cas_rn=112055-76-2 | |
Description | CAS Common Chemistry is an open community resource for accessing chemical information. Nearly 500,000 chemical substances from CAS REGISTRY cover areas of community interest, including common and frequently regulated chemicals, and those relevant to high school and undergraduate chemistry classes. This chemical information, curated by our expert scientists, is provided in alignment with our mission as a division of the American Chemical Society. | |
Explanation | The data from CAS Common Chemistry is provided under a CC-BY-NC 4.0 license, unless otherwise stated. | |
Record name | Decylplastoquinone | |
Source | EPA DSSTox | |
URL | https://comptox.epa.gov/dashboard/DTXSID60409010 | |
Description | DSSTox provides a high quality public chemistry resource for supporting improved predictive toxicology. | |
Record name | Decylplastoquinone | |
Source | European Chemicals Agency (ECHA) | |
URL | https://echa.europa.eu/information-on-chemicals | |
Description | The European Chemicals Agency (ECHA) is an agency of the European Union which is the driving force among regulatory authorities in implementing the EU's groundbreaking chemicals legislation for the benefit of human health and the environment as well as for innovation and competitiveness. | |
Explanation | Use of the information, documents and data from the ECHA website is subject to the terms and conditions of this Legal Notice, and subject to other binding limitations provided for under applicable law, the information, documents and data made available on the ECHA website may be reproduced, distributed and/or used, totally or in part, for non-commercial purposes provided that ECHA is acknowledged as the source: "Source: European Chemicals Agency, http://echa.europa.eu/". Such acknowledgement must be included in each copy of the material. ECHA permits and encourages organisations and individuals to create links to the ECHA website under the following cumulative conditions: Links can only be made to webpages that provide a link to the Legal Notice page. | |
Retrosynthesis Analysis
AI-Powered Synthesis Planning: Our tool employs the Template_relevance Pistachio, Template_relevance Bkms_metabolic, Template_relevance Pistachio_ringbreaker, Template_relevance Reaxys, Template_relevance Reaxys_biocatalysis model, leveraging a vast database of chemical reactions to predict feasible synthetic routes.
One-Step Synthesis Focus: Specifically designed for one-step synthesis, it provides concise and direct routes for your target compounds, streamlining the synthesis process.
Accurate Predictions: Utilizing the extensive PISTACHIO, BKMS_METABOLIC, PISTACHIO_RINGBREAKER, REAXYS, REAXYS_BIOCATALYSIS database, our tool offers high-accuracy predictions, reflecting the latest in chemical research and data.
Strategy Settings
Precursor scoring | Relevance Heuristic |
---|---|
Min. plausibility | 0.01 |
Model | Template_relevance |
Template Set | Pistachio/Bkms_metabolic/Pistachio_ringbreaker/Reaxys/Reaxys_biocatalysis |
Top-N result to add to graph | 6 |
Feasible Synthetic Routes
Disclaimer and Information on In-Vitro Research Products
Please be aware that all articles and product information presented on BenchChem are intended solely for informational purposes. The products available for purchase on BenchChem are specifically designed for in-vitro studies, which are conducted outside of living organisms. In-vitro studies, derived from the Latin term "in glass," involve experiments performed in controlled laboratory settings using cells or tissues. It is important to note that these products are not categorized as medicines or drugs, and they have not received approval from the FDA for the prevention, treatment, or cure of any medical condition, ailment, or disease. We must emphasize that any form of bodily introduction of these products into humans or animals is strictly prohibited by law. It is essential to adhere to these guidelines to ensure compliance with legal and ethical standards in research and experimentation.