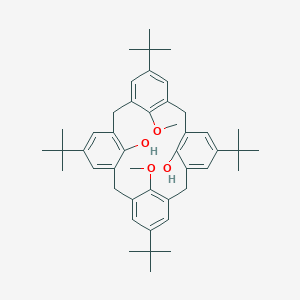
1,3-Dimethoxy-4-tert-butylcalix(4)arene
Overview
Description
1,3-Dimethoxy-4-tert-butylcalix(4)arene is a derivative of calixarene, a type of macrocyclic compound with a phenolic backbone. Calixarenes are known for their ability to form host-guest complexes with various molecules and ions, making them valuable in supramolecular chemistry. The tert-butyl groups at the 4-position and methoxy groups at the 1,3-positions enhance the compound’s solubility and stability, making it suitable for various applications.
Mechanism of Action
Target of Action
The primary targets of 1,3-Dimethoxy-4-tert-butylcalix(4)arene are organomagnesium reagents and zinc bromide . These targets play a crucial role in the formation of mixed-metal calix4arene systems .
Mode of Action
This compound interacts with its targets through a reaction process. Different combinations of organomagnesium reagents and zinc bromide react with this compound to afford mixed-metal calix4arene systems . This interaction results in the formation of intriguing molecular structures .
Biochemical Pathways
The affected pathway involves the ring-opening polymerisation of ε-caprolactone . The downstream effects of this pathway include the formation of intriguing molecular structures and the capability of the systems to undergo ring-opening polymerisation under N2, air, or as melts .
Pharmacokinetics
The compound’s interaction with organomagnesium reagents and zinc bromide suggests that it may have significant bioavailability in systems where these targets are present .
Result of Action
The result of the action of this compound is the formation of mixed-metal calix4arene systems . These systems have intriguing molecular structures and are capable of the ring-opening polymerisation of ε-caprolactone .
Action Environment
The action of this compound can be influenced by environmental factors such as the presence of organomagnesium reagents and zinc bromide . These factors can affect the compound’s action, efficacy, and stability .
Biochemical Analysis
Biochemical Properties
Different combinations of organomagnesium reagents and zinc bromide react with 1,3-Dimethoxy-4-tert-butylcalix(4)arene to afford mixed-metal calix4arene systems . These systems are capable of the ring opening polymerisation of ε-caprolactone under N2, air, or as melts .
Cellular Effects
arene derivatives have been shown to exhibit cytotoxic activity against different human cancer cells.
Molecular Mechanism
The molecular mechanism of this compound is not fully understood. It is known that calix4arene systems can form intriguing molecular structures .
Preparation Methods
Synthetic Routes and Reaction Conditions
1,3-Dimethoxy-4-tert-butylcalix(4)arene can be synthesized through a multi-step process involving the following steps:
Formation of the calixarene backbone: The initial step involves the condensation of p-tert-butylphenol with formaldehyde under basic conditions to form p-tert-butylcalix(4)arene.
Industrial Production Methods
Industrial production methods for this compound typically involve optimizing the reaction conditions to maximize yield and purity. This includes controlling the temperature, reaction time, and the molar ratios of reactants. The use of continuous flow reactors and automated systems can further enhance the efficiency and scalability of the production process .
Chemical Reactions Analysis
Types of Reactions
1,3-Dimethoxy-4-tert-butylcalix(4)arene undergoes various chemical reactions, including:
Substitution Reactions: The methoxy groups can be substituted with other functional groups using appropriate reagents.
Complexation Reactions: The compound can form complexes with metal ions and organic molecules through host-guest interactions.
Common Reagents and Conditions
Substitution Reactions: Reagents such as halides and bases are commonly used for substitution reactions.
Complexation Reactions: Metal salts and organic molecules are used to form complexes under mild conditions.
Major Products
Substitution Products: Depending on the substituent introduced, various functionalized calixarenes can be obtained.
Complexes: Metal-calixarene complexes and organic molecule-calixarene complexes are the major products.
Scientific Research Applications
1,3-Dimethoxy-4-tert-butylcalix(4)arene has a wide range of scientific research applications, including:
Comparison with Similar Compounds
Similar Compounds
p-tert-Butylcalix(4)arene: Lacks the methoxy groups, resulting in different solubility and complexation properties.
1,3-Dihydroxy-4-tert-butylcalix(4)arene: Contains hydroxyl groups instead of methoxy groups, affecting its reactivity and complexation behavior.
Uniqueness
1,3-Dimethoxy-4-tert-butylcalix(4)arene is unique due to its enhanced solubility and stability, which make it more suitable for applications requiring these properties.
Biological Activity
Overview
1,3-Dimethoxy-4-tert-butylcalix(4)arene is a member of the calixarene family, known for its unique structural properties and biological activities. This compound exhibits significant potential in various fields including medicinal chemistry, supramolecular chemistry, and materials science. The presence of methoxy and tert-butyl groups enhances its solubility and stability, making it an interesting candidate for biological applications.
Antimicrobial Activity
This compound has been studied for its antimicrobial properties. Research indicates that it shows notable activity against various bacterial strains, particularly Gram-positive bacteria. For example, derivatives of calixarenes have demonstrated effectiveness against methicillin-resistant Staphylococcus aureus (MRSA) and other pathogenic bacteria .
Compound | Bacterial Strain | Minimum Inhibitory Concentration (MIC) |
---|---|---|
This compound | MRSA | 13–25 µg/ml |
Quaternary ammonium salt derivative | MRSA | 3.1 µg/ml |
This table summarizes the MIC values for different derivatives of calixarene compounds against MRSA, highlighting the enhanced activity of quaternary ammonium salt substitutions.
Cytotoxicity
In addition to its antimicrobial properties, this compound has shown cytotoxic effects on various human cancer cell lines. Its mechanism appears to involve disruption of cellular membranes and interference with cellular processes . Research has indicated that calixarene derivatives can induce apoptosis in cancer cells through various biochemical pathways.
The biological activity of this compound is attributed to its ability to form host-guest complexes with various ions and molecules. This interaction is facilitated by the unique structural features of the calixarene scaffold, allowing it to selectively bind to target species. The interaction with organomagnesium reagents and zinc bromide suggests potential applications in drug delivery systems due to enhanced bioavailability .
Cellular Effects
The compound’s interactions can lead to significant changes in cellular behavior:
- Membrane Disruption : The amphiphilic nature of calixarene derivatives allows them to insert into lipid membranes, leading to cell lysis.
- Apoptosis Induction : Certain derivatives have been shown to activate apoptotic pathways in cancer cells.
Study on Antibacterial Activity
A study evaluated the antibacterial activity of several calixarene derivatives including this compound against multiple bacterial strains. The results indicated that while some derivatives exhibited strong antibacterial properties, others showed limited activity depending on their structural modifications .
Cytotoxicity Assessment
In another investigation focusing on cytotoxicity, researchers assessed the effects of this compound on human cancer cell lines. The findings revealed that the compound induced significant cytotoxic effects at micromolar concentrations, highlighting its potential as a chemotherapeutic agent .
Properties
IUPAC Name |
5,11,17,23-tetratert-butyl-26,28-dimethoxypentacyclo[19.3.1.13,7.19,13.115,19]octacosa-1(24),3,5,7(28),9,11,13(27),15(26),16,18,21(25),22-dodecaene-25,27-diol | |
---|---|---|
Source | PubChem | |
URL | https://pubchem.ncbi.nlm.nih.gov | |
Description | Data deposited in or computed by PubChem | |
InChI |
InChI=1S/C46H60O4/c1-43(2,3)35-19-27-15-31-23-37(45(7,8)9)25-33(41(31)49-13)17-29-21-36(44(4,5)6)22-30(40(29)48)18-34-26-38(46(10,11)12)24-32(42(34)50-14)16-28(20-35)39(27)47/h19-26,47-48H,15-18H2,1-14H3 | |
Source | PubChem | |
URL | https://pubchem.ncbi.nlm.nih.gov | |
Description | Data deposited in or computed by PubChem | |
InChI Key |
SDNQRJYYUPMBIV-UHFFFAOYSA-N | |
Source | PubChem | |
URL | https://pubchem.ncbi.nlm.nih.gov | |
Description | Data deposited in or computed by PubChem | |
Canonical SMILES |
CC(C)(C)C1=CC2=C(C(=C1)CC3=CC(=CC(=C3OC)CC4=CC(=CC(=C4O)CC5=C(C(=CC(=C5)C(C)(C)C)C2)OC)C(C)(C)C)C(C)(C)C)O | |
Source | PubChem | |
URL | https://pubchem.ncbi.nlm.nih.gov | |
Description | Data deposited in or computed by PubChem | |
Molecular Formula |
C46H60O4 | |
Source | PubChem | |
URL | https://pubchem.ncbi.nlm.nih.gov | |
Description | Data deposited in or computed by PubChem | |
Molecular Weight |
677.0 g/mol | |
Source | PubChem | |
URL | https://pubchem.ncbi.nlm.nih.gov | |
Description | Data deposited in or computed by PubChem | |
Retrosynthesis Analysis
AI-Powered Synthesis Planning: Our tool employs the Template_relevance Pistachio, Template_relevance Bkms_metabolic, Template_relevance Pistachio_ringbreaker, Template_relevance Reaxys, Template_relevance Reaxys_biocatalysis model, leveraging a vast database of chemical reactions to predict feasible synthetic routes.
One-Step Synthesis Focus: Specifically designed for one-step synthesis, it provides concise and direct routes for your target compounds, streamlining the synthesis process.
Accurate Predictions: Utilizing the extensive PISTACHIO, BKMS_METABOLIC, PISTACHIO_RINGBREAKER, REAXYS, REAXYS_BIOCATALYSIS database, our tool offers high-accuracy predictions, reflecting the latest in chemical research and data.
Strategy Settings
Precursor scoring | Relevance Heuristic |
---|---|
Min. plausibility | 0.01 |
Model | Template_relevance |
Template Set | Pistachio/Bkms_metabolic/Pistachio_ringbreaker/Reaxys/Reaxys_biocatalysis |
Top-N result to add to graph | 6 |
Feasible Synthetic Routes
Disclaimer and Information on In-Vitro Research Products
Please be aware that all articles and product information presented on BenchChem are intended solely for informational purposes. The products available for purchase on BenchChem are specifically designed for in-vitro studies, which are conducted outside of living organisms. In-vitro studies, derived from the Latin term "in glass," involve experiments performed in controlled laboratory settings using cells or tissues. It is important to note that these products are not categorized as medicines or drugs, and they have not received approval from the FDA for the prevention, treatment, or cure of any medical condition, ailment, or disease. We must emphasize that any form of bodily introduction of these products into humans or animals is strictly prohibited by law. It is essential to adhere to these guidelines to ensure compliance with legal and ethical standards in research and experimentation.