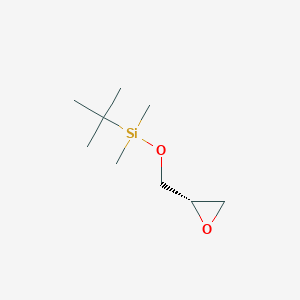
tert-Butyldimethylsilyl (S)-(+)-glycidyl ether
Overview
Description
tert-Butyldimethylsilyl (S)-(+)-glycidyl ether is an organosilicon compound widely used in organic synthesis. It is known for its role as a protecting group for alcohols, which helps to prevent unwanted reactions during complex synthetic procedures. The compound’s structure includes a glycidyl ether moiety, which is an epoxide, and a tert-butyldimethylsilyl group, which provides steric protection.
Mechanism of Action
Target of Action
It is known that this compound is often used in organic synthesis as a protecting group for alcohols .
Mode of Action
The mode of action of tert-Butyldimethylsilyl (S)-(+)-glycidyl ether involves its reaction with alcohols to form silyl ethers . This reaction is catalyzed by dimethylformamide (DMF) and results in the conversion of various alcohols to tert-butyldimethylsilyl ethers . The silyl ether group serves as a protective group, preventing the alcohol from reacting under conditions that would normally promote such reactions .
Biochemical Pathways
The compound plays a crucial role in the synthesis of complex organic molecules, where it protects reactive alcohol groups from unwanted reactions during the synthetic process .
Result of Action
The primary result of the action of this compound is the formation of silyl ethers, which serve as protective groups for alcohols in organic synthesis . These silyl ethers can be easily deprotected by employing a catalytic amount of acetyl chloride in dry methanol, yielding the original alcohol .
Action Environment
The action of this compound is influenced by several environmental factors. For instance, the reaction of this compound with alcohols to form silyl ethers is catalyzed by DMF . Additionally, the deprotection of the silyl ethers to yield the original alcohols can be achieved using acetyl chloride in dry methanol . The reaction conditions, such as temperature and solvent, can significantly influence the efficiency and yield of these reactions.
Biochemical Analysis
Biochemical Properties
The compound plays a role in biochemical reactions as a protecting group for alcohols . It interacts with enzymes and other biomolecules during the process of silylation, where it forms a covalent bond with the hydroxyl group of an alcohol . The nature of these interactions is largely determined by the steric bulk and electronic properties of the tert-Butyldimethylsilyl (S)-(+)-glycidyl ether .
Cellular Effects
As a silyl ether, it may influence cell function by modifying the chemical properties of biomolecules, potentially impacting cell signaling pathways, gene expression, and cellular metabolism .
Molecular Mechanism
The molecular mechanism of action of this compound involves the formation and cleavage of silyl ethers . The compound can form a covalent bond with the hydroxyl group of an alcohol, creating a silyl ether that is resistant to hydrolysis . This bond can be cleaved under certain conditions, releasing the protected alcohol .
Temporal Effects in Laboratory Settings
In laboratory settings, the effects of this compound can change over time due to its reactivity. The compound is stable under basic conditions but can be hydrolyzed back to the alcohol under acidic conditions . The stability and degradation of the compound can influence its long-term effects on cellular function .
Metabolic Pathways
As a silyl ether, it may interact with enzymes or cofactors involved in the metabolism of alcohols .
Transport and Distribution
As a small, lipophilic molecule, it may diffuse across cell membranes and distribute throughout the cell .
Subcellular Localization
As a small, lipophilic molecule, it may localize to various compartments or organelles within the cell .
Preparation Methods
Synthetic Routes and Reaction Conditions
tert-Butyldimethylsilyl (S)-(+)-glycidyl ether can be synthesized from glycidol through a silylation reaction. The process involves the reaction of glycidol with tert-butyldimethylsilyl chloride in the presence of a base such as imidazole or pyridine. The reaction is typically carried out in an organic solvent like dimethylformamide or acetonitrile at room temperature to slightly elevated temperatures .
Industrial Production Methods
In industrial settings, the production of this compound follows similar principles but on a larger scale. The use of continuous flow reactors and optimized reaction conditions ensures high yield and purity. The process involves careful control of temperature, pressure, and reactant concentrations to achieve efficient production.
Chemical Reactions Analysis
Types of Reactions
tert-Butyldimethylsilyl (S)-(+)-glycidyl ether undergoes various chemical reactions, including:
Oxidation: The epoxide ring can be opened through oxidation reactions.
Reduction: The compound can be reduced to form different alcohol derivatives.
Substitution: The silyl ether group can be substituted under acidic or basic conditions.
Common Reagents and Conditions
Oxidation: Reagents like potassium permanganate or osmium tetroxide can be used.
Reduction: Lithium aluminum hydride or sodium borohydride are common reducing agents.
Substitution: Acidic conditions (e.g., acetic acid) or basic conditions (e.g., sodium hydroxide) can facilitate the substitution reactions.
Major Products Formed
The major products formed from these reactions include various alcohols, ethers, and other derivatives depending on the specific reaction conditions and reagents used.
Scientific Research Applications
tert-Butyldimethylsilyl (S)-(+)-glycidyl ether is used in a wide range of scientific research applications:
Chemistry: It serves as a protecting group for alcohols in multi-step organic syntheses, allowing for selective reactions to occur without interference from hydroxyl groups.
Biology: The compound is used in the synthesis of biologically active molecules, including pharmaceuticals and agrochemicals.
Medicine: It is involved in the development of drug candidates and the modification of biomolecules for therapeutic purposes.
Industry: The compound is used in the production of specialty chemicals, polymers, and advanced materials
Comparison with Similar Compounds
Similar Compounds
Trimethylsilyl ether: Less sterically hindered and more susceptible to hydrolysis.
tert-Butyldiphenylsilyl ether: Provides greater steric protection and stability under acidic conditions.
Triisopropylsilyl ether: Offers a balance between steric protection and ease of removal.
Uniqueness
tert-Butyldimethylsilyl (S)-(+)-glycidyl ether is unique due to its combination of steric protection and reactivity. It provides a good balance between stability and ease of removal, making it a versatile protecting group in organic synthesis. Its ability to protect hydroxyl groups under a wide range of conditions sets it apart from other silyl ethers .
Biological Activity
tert-Butyldimethylsilyl (S)-(+)-glycidyl ether (TBDMS-glycidyl ether) is a chemical compound widely utilized in organic synthesis, particularly as a protecting group for hydroxyl functionalities. Its unique structure imparts specific reactivity and biological activity, making it a subject of interest in medicinal chemistry and polymer science. This article delves into the biological activity of TBDMS-glycidyl ether, highlighting its applications, mechanisms of action, and relevant case studies.
- Molecular Formula : C₉H₂₀O₂Si
- Molecular Weight : 188.34 g/mol
- CAS Number : 123237-62-7
- Boiling Point : 30 °C
- Flash Point : 73 °C
The TBDMS group in TBDMS-glycidyl ether acts as a protective entity for hydroxyl groups, enabling selective reactions without interference from the hydroxyl's inherent reactivity. This property is particularly advantageous in multi-step synthetic pathways where the preservation of certain functional groups is crucial.
Biological Activity Overview
Research indicates that TBDMS-glycidyl ether exhibits various biological activities, primarily through its role as a reactive intermediate in organic synthesis. Its ability to form stable intermediates makes it valuable in the development of pharmaceutical compounds.
Key Biological Activities:
- Antitubercular Activity : Preliminary studies suggest that derivatives of TBDMS-glycidyl ether may exhibit antitubercular properties, akin to other glycidyl ethers that have shown efficacy against Mycobacterium tuberculosis .
- Silylation Reactions : The compound facilitates silylation reactions, enhancing the stability and reactivity of alcohols and phenols during synthetic processes.
- Polymer Chemistry Applications : It plays a significant role in modifying molecular structures in polymer chemistry, contributing to the development of novel materials with enhanced properties.
Case Study 1: Antitubercular Activity
A study investigated the synthesis of various glycidyl silyl ethers, including TBDMS-glycidyl ether, and their biological evaluation against Mycobacterium tuberculosis. The results indicated that certain derivatives exhibited significant antitubercular activity, suggesting potential therapeutic applications .
Case Study 2: Silylation Efficiency
Research focused on the efficiency of TBDMS-glycidyl ether as a silylating agent demonstrated its effectiveness in protecting hydroxyl groups under mild conditions. The study highlighted the compound's ability to enhance selectivity during multi-step reactions, thereby improving overall yields.
Data Table: Comparison of Biological Activities
Properties
IUPAC Name |
tert-butyl-dimethyl-[[(2S)-oxiran-2-yl]methoxy]silane | |
---|---|---|
Source | PubChem | |
URL | https://pubchem.ncbi.nlm.nih.gov | |
Description | Data deposited in or computed by PubChem | |
InChI |
InChI=1S/C9H20O2Si/c1-9(2,3)12(4,5)11-7-8-6-10-8/h8H,6-7H2,1-5H3/t8-/m0/s1 | |
Source | PubChem | |
URL | https://pubchem.ncbi.nlm.nih.gov | |
Description | Data deposited in or computed by PubChem | |
InChI Key |
YANSSVVGZPNSKD-QMMMGPOBSA-N | |
Source | PubChem | |
URL | https://pubchem.ncbi.nlm.nih.gov | |
Description | Data deposited in or computed by PubChem | |
Canonical SMILES |
CC(C)(C)[Si](C)(C)OCC1CO1 | |
Source | PubChem | |
URL | https://pubchem.ncbi.nlm.nih.gov | |
Description | Data deposited in or computed by PubChem | |
Isomeric SMILES |
CC(C)(C)[Si](C)(C)OC[C@@H]1CO1 | |
Source | PubChem | |
URL | https://pubchem.ncbi.nlm.nih.gov | |
Description | Data deposited in or computed by PubChem | |
Molecular Formula |
C9H20O2Si | |
Source | PubChem | |
URL | https://pubchem.ncbi.nlm.nih.gov | |
Description | Data deposited in or computed by PubChem | |
DSSTOX Substance ID |
DTXSID80449610 | |
Record name | tert-Butyl(dimethyl){[(2S)-oxiran-2-yl]methoxy}silane | |
Source | EPA DSSTox | |
URL | https://comptox.epa.gov/dashboard/DTXSID80449610 | |
Description | DSSTox provides a high quality public chemistry resource for supporting improved predictive toxicology. | |
Molecular Weight |
188.34 g/mol | |
Source | PubChem | |
URL | https://pubchem.ncbi.nlm.nih.gov | |
Description | Data deposited in or computed by PubChem | |
CAS No. |
123237-62-7 | |
Record name | tert-Butyldimethyl[((S)-oxiranyl)methoxy]silane | |
Source | CAS Common Chemistry | |
URL | https://commonchemistry.cas.org/detail?cas_rn=123237-62-7 | |
Description | CAS Common Chemistry is an open community resource for accessing chemical information. Nearly 500,000 chemical substances from CAS REGISTRY cover areas of community interest, including common and frequently regulated chemicals, and those relevant to high school and undergraduate chemistry classes. This chemical information, curated by our expert scientists, is provided in alignment with our mission as a division of the American Chemical Society. | |
Explanation | The data from CAS Common Chemistry is provided under a CC-BY-NC 4.0 license, unless otherwise stated. | |
Record name | tert-Butyl(dimethyl){[(2S)-oxiran-2-yl]methoxy}silane | |
Source | EPA DSSTox | |
URL | https://comptox.epa.gov/dashboard/DTXSID80449610 | |
Description | DSSTox provides a high quality public chemistry resource for supporting improved predictive toxicology. | |
Synthesis routes and methods I
Procedure details
Synthesis routes and methods II
Procedure details
Retrosynthesis Analysis
AI-Powered Synthesis Planning: Our tool employs the Template_relevance Pistachio, Template_relevance Bkms_metabolic, Template_relevance Pistachio_ringbreaker, Template_relevance Reaxys, Template_relevance Reaxys_biocatalysis model, leveraging a vast database of chemical reactions to predict feasible synthetic routes.
One-Step Synthesis Focus: Specifically designed for one-step synthesis, it provides concise and direct routes for your target compounds, streamlining the synthesis process.
Accurate Predictions: Utilizing the extensive PISTACHIO, BKMS_METABOLIC, PISTACHIO_RINGBREAKER, REAXYS, REAXYS_BIOCATALYSIS database, our tool offers high-accuracy predictions, reflecting the latest in chemical research and data.
Strategy Settings
Precursor scoring | Relevance Heuristic |
---|---|
Min. plausibility | 0.01 |
Model | Template_relevance |
Template Set | Pistachio/Bkms_metabolic/Pistachio_ringbreaker/Reaxys/Reaxys_biocatalysis |
Top-N result to add to graph | 6 |
Feasible Synthetic Routes
Disclaimer and Information on In-Vitro Research Products
Please be aware that all articles and product information presented on BenchChem are intended solely for informational purposes. The products available for purchase on BenchChem are specifically designed for in-vitro studies, which are conducted outside of living organisms. In-vitro studies, derived from the Latin term "in glass," involve experiments performed in controlled laboratory settings using cells or tissues. It is important to note that these products are not categorized as medicines or drugs, and they have not received approval from the FDA for the prevention, treatment, or cure of any medical condition, ailment, or disease. We must emphasize that any form of bodily introduction of these products into humans or animals is strictly prohibited by law. It is essential to adhere to these guidelines to ensure compliance with legal and ethical standards in research and experimentation.