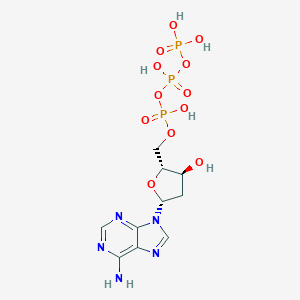
2'-Deoxyadenosine 5'-triphosphate
Overview
Description
2'-Deoxyadenosine 5'-triphosphate (dATP) is a nucleotide that plays a crucial role in DNA synthesis and repair. It is a building block of DNA and is involved in the transfer of energy in biochemical reactions.
Scientific Research Applications
Improved Synthesis and Analogs as Drugs
2'-Deoxyadenosine 5'-triphosphate (dATP) synthesis has been enhanced with methods involving overexpressing enzymes in Escherichia coli and gram-scale chemical synthesis. It is involved in studies related to DNA damage, nucleotide pool sanitization, and translesion synthesis. Purine nucleoside analogs of dATP have been used in treating chronic lymphoid leukemias, and certain analogs have shown promise in delaying polymerization arrest during HIV-1 reverse transcription (Biswas, Kar, & Chattopadhyaya, 2013).
Concise and Efficient Synthesis
A one-pot synthetic methodology successfully produced 2'-deoxyadenosine-3'-O-triphosphate (d-3'-ATP) with a high yield, demonstrating its various biological applications (Kore, Yang, & Srinivasan, 2014).
Biological Applications in DNA Synthesis and Modification
Hydrophobic DNA Modifications
Modifications of 2'-deoxyadenosine, like the additions of alkyl- or arylthiols to 7-vinyl-7-deaza-2'-deoxyadenosine, produced a series of modified nucleosides and triphosphates. These modified triphosphates were substrates for DNA polymerases, used in the enzymatic synthesis of base-modified oligonucleotides or DNA containing hydrophobic substituents (Slavíčková, Pohl, & Hocek, 2016).
Minor Groove DNA Modifications
The study explored the substrate properties of modified derivatives of dATP during enzymatic reactions. This led to the enzymatic preparation of modified DNA, potentially enhancing its physicochemical properties. The Vent (exo-) DNA polymerase was found most effective for the modified substrates (Volkova, Chudinov, & Lapa, 2021).
Quantitative Analysis and Bioorthogonal Labeling
Quantitative Analysis in Cells
Liquid chromatography tandem mass spectrometry methods were developed to quantify nucleosides and nucleoside triphosphates, including dATP, from cells. This methodology aids in understanding the metabolic pathways and functions of these nucleotides in various cellular processes (Thomas et al., 2015).
Bioorthogonal Labeling
Modified dATP analogues were synthesized for bioorthogonal labeling in DNA synthesis. The real-time kinetic data revealed faster incorporation of modified dATP into DNA compared to similar analogues, demonstrating its application in site-specific labeling and visualization in living cells (Ploschik et al., 2018).
properties
IUPAC Name |
[[(2R,3S,5R)-5-(6-aminopurin-9-yl)-3-hydroxyoxolan-2-yl]methoxy-hydroxyphosphoryl] phosphono hydrogen phosphate | |
---|---|---|
Source | PubChem | |
URL | https://pubchem.ncbi.nlm.nih.gov | |
Description | Data deposited in or computed by PubChem | |
InChI |
InChI=1S/C10H16N5O12P3/c11-9-8-10(13-3-12-9)15(4-14-8)7-1-5(16)6(25-7)2-24-29(20,21)27-30(22,23)26-28(17,18)19/h3-7,16H,1-2H2,(H,20,21)(H,22,23)(H2,11,12,13)(H2,17,18,19)/t5-,6+,7+/m0/s1 | |
Source | PubChem | |
URL | https://pubchem.ncbi.nlm.nih.gov | |
Description | Data deposited in or computed by PubChem | |
InChI Key |
SUYVUBYJARFZHO-RRKCRQDMSA-N | |
Source | PubChem | |
URL | https://pubchem.ncbi.nlm.nih.gov | |
Description | Data deposited in or computed by PubChem | |
Canonical SMILES |
C1C(C(OC1N2C=NC3=C(N=CN=C32)N)COP(=O)(O)OP(=O)(O)OP(=O)(O)O)O | |
Source | PubChem | |
URL | https://pubchem.ncbi.nlm.nih.gov | |
Description | Data deposited in or computed by PubChem | |
Isomeric SMILES |
C1[C@@H]([C@H](O[C@H]1N2C=NC3=C(N=CN=C32)N)COP(=O)(O)OP(=O)(O)OP(=O)(O)O)O | |
Source | PubChem | |
URL | https://pubchem.ncbi.nlm.nih.gov | |
Description | Data deposited in or computed by PubChem | |
Molecular Formula |
C10H16N5O12P3 | |
Source | PubChem | |
URL | https://pubchem.ncbi.nlm.nih.gov | |
Description | Data deposited in or computed by PubChem | |
DSSTOX Substance ID |
DTXSID10895848 | |
Record name | 2'-Deoxyadenosine triphosphate | |
Source | EPA DSSTox | |
URL | https://comptox.epa.gov/dashboard/DTXSID10895848 | |
Description | DSSTox provides a high quality public chemistry resource for supporting improved predictive toxicology. | |
Molecular Weight |
491.18 g/mol | |
Source | PubChem | |
URL | https://pubchem.ncbi.nlm.nih.gov | |
Description | Data deposited in or computed by PubChem | |
Physical Description |
Solid | |
Record name | Deoxyadenosine triphosphate | |
Source | Human Metabolome Database (HMDB) | |
URL | http://www.hmdb.ca/metabolites/HMDB0001532 | |
Description | The Human Metabolome Database (HMDB) is a freely available electronic database containing detailed information about small molecule metabolites found in the human body. | |
Explanation | HMDB is offered to the public as a freely available resource. Use and re-distribution of the data, in whole or in part, for commercial purposes requires explicit permission of the authors and explicit acknowledgment of the source material (HMDB) and the original publication (see the HMDB citing page). We ask that users who download significant portions of the database cite the HMDB paper in any resulting publications. | |
CAS RN |
1927-31-7, 67460-17-7 | |
Record name | dATP | |
Source | CAS Common Chemistry | |
URL | https://commonchemistry.cas.org/detail?cas_rn=1927-31-7 | |
Description | CAS Common Chemistry is an open community resource for accessing chemical information. Nearly 500,000 chemical substances from CAS REGISTRY cover areas of community interest, including common and frequently regulated chemicals, and those relevant to high school and undergraduate chemistry classes. This chemical information, curated by our expert scientists, is provided in alignment with our mission as a division of the American Chemical Society. | |
Explanation | The data from CAS Common Chemistry is provided under a CC-BY-NC 4.0 license, unless otherwise stated. | |
Record name | 2'-Deoxyadenosine triphosphate | |
Source | ChemIDplus | |
URL | https://pubchem.ncbi.nlm.nih.gov/substance/?source=chemidplus&sourceid=0001927317 | |
Description | ChemIDplus is a free, web search system that provides access to the structure and nomenclature authority files used for the identification of chemical substances cited in National Library of Medicine (NLM) databases, including the TOXNET system. | |
Record name | 2'-Deoxyformycin-5'-triphosphate | |
Source | ChemIDplus | |
URL | https://pubchem.ncbi.nlm.nih.gov/substance/?source=chemidplus&sourceid=0067460177 | |
Description | ChemIDplus is a free, web search system that provides access to the structure and nomenclature authority files used for the identification of chemical substances cited in National Library of Medicine (NLM) databases, including the TOXNET system. | |
Record name | dATP | |
Source | DrugBank | |
URL | https://www.drugbank.ca/drugs/DB03222 | |
Description | The DrugBank database is a unique bioinformatics and cheminformatics resource that combines detailed drug (i.e. chemical, pharmacological and pharmaceutical) data with comprehensive drug target (i.e. sequence, structure, and pathway) information. | |
Explanation | Creative Common's Attribution-NonCommercial 4.0 International License (http://creativecommons.org/licenses/by-nc/4.0/legalcode) | |
Record name | 2'-Deoxyadenosine triphosphate | |
Source | EPA DSSTox | |
URL | https://comptox.epa.gov/dashboard/DTXSID10895848 | |
Description | DSSTox provides a high quality public chemistry resource for supporting improved predictive toxicology. | |
Record name | 2'-deoxyadenosine 5'-(tetrahydrogen triphosphate) | |
Source | European Chemicals Agency (ECHA) | |
URL | https://echa.europa.eu/substance-information/-/substanceinfo/100.016.058 | |
Description | The European Chemicals Agency (ECHA) is an agency of the European Union which is the driving force among regulatory authorities in implementing the EU's groundbreaking chemicals legislation for the benefit of human health and the environment as well as for innovation and competitiveness. | |
Explanation | Use of the information, documents and data from the ECHA website is subject to the terms and conditions of this Legal Notice, and subject to other binding limitations provided for under applicable law, the information, documents and data made available on the ECHA website may be reproduced, distributed and/or used, totally or in part, for non-commercial purposes provided that ECHA is acknowledged as the source: "Source: European Chemicals Agency, http://echa.europa.eu/". Such acknowledgement must be included in each copy of the material. ECHA permits and encourages organisations and individuals to create links to the ECHA website under the following cumulative conditions: Links can only be made to webpages that provide a link to the Legal Notice page. | |
Record name | 2'-DEOXYADENOSINE TRIPHOSPHATE | |
Source | FDA Global Substance Registration System (GSRS) | |
URL | https://gsrs.ncats.nih.gov/ginas/app/beta/substances/K8KCC8SH6N | |
Description | The FDA Global Substance Registration System (GSRS) enables the efficient and accurate exchange of information on what substances are in regulated products. Instead of relying on names, which vary across regulatory domains, countries, and regions, the GSRS knowledge base makes it possible for substances to be defined by standardized, scientific descriptions. | |
Explanation | Unless otherwise noted, the contents of the FDA website (www.fda.gov), both text and graphics, are not copyrighted. They are in the public domain and may be republished, reprinted and otherwise used freely by anyone without the need to obtain permission from FDA. Credit to the U.S. Food and Drug Administration as the source is appreciated but not required. | |
Record name | Deoxyadenosine triphosphate | |
Source | Human Metabolome Database (HMDB) | |
URL | http://www.hmdb.ca/metabolites/HMDB0001532 | |
Description | The Human Metabolome Database (HMDB) is a freely available electronic database containing detailed information about small molecule metabolites found in the human body. | |
Explanation | HMDB is offered to the public as a freely available resource. Use and re-distribution of the data, in whole or in part, for commercial purposes requires explicit permission of the authors and explicit acknowledgment of the source material (HMDB) and the original publication (see the HMDB citing page). We ask that users who download significant portions of the database cite the HMDB paper in any resulting publications. | |
Retrosynthesis Analysis
AI-Powered Synthesis Planning: Our tool employs the Template_relevance Pistachio, Template_relevance Bkms_metabolic, Template_relevance Pistachio_ringbreaker, Template_relevance Reaxys, Template_relevance Reaxys_biocatalysis model, leveraging a vast database of chemical reactions to predict feasible synthetic routes.
One-Step Synthesis Focus: Specifically designed for one-step synthesis, it provides concise and direct routes for your target compounds, streamlining the synthesis process.
Accurate Predictions: Utilizing the extensive PISTACHIO, BKMS_METABOLIC, PISTACHIO_RINGBREAKER, REAXYS, REAXYS_BIOCATALYSIS database, our tool offers high-accuracy predictions, reflecting the latest in chemical research and data.
Strategy Settings
Precursor scoring | Relevance Heuristic |
---|---|
Min. plausibility | 0.01 |
Model | Template_relevance |
Template Set | Pistachio/Bkms_metabolic/Pistachio_ringbreaker/Reaxys/Reaxys_biocatalysis |
Top-N result to add to graph | 6 |
Feasible Synthetic Routes
Disclaimer and Information on In-Vitro Research Products
Please be aware that all articles and product information presented on BenchChem are intended solely for informational purposes. The products available for purchase on BenchChem are specifically designed for in-vitro studies, which are conducted outside of living organisms. In-vitro studies, derived from the Latin term "in glass," involve experiments performed in controlled laboratory settings using cells or tissues. It is important to note that these products are not categorized as medicines or drugs, and they have not received approval from the FDA for the prevention, treatment, or cure of any medical condition, ailment, or disease. We must emphasize that any form of bodily introduction of these products into humans or animals is strictly prohibited by law. It is essential to adhere to these guidelines to ensure compliance with legal and ethical standards in research and experimentation.