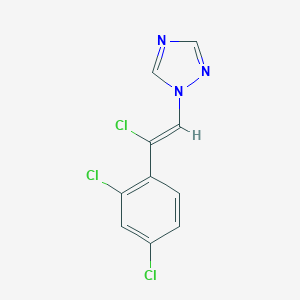
Loreclezole
Overview
Description
Loreclezole is a positive allosteric modulator (PAM) of γ-aminobutyric acid type A (GABAA) receptors, initially developed as an anticonvulsant . Structurally, it contains a 1,2,4-triazole ring with a chlorine substituent, a scaffold known to enhance biological activity through halogen interactions . Its mechanism involves selective enhancement of GABAA receptors containing β2 or β3 subunits, driven by a critical asparagine residue (β2Asn265 or β3Asn265) in the transmembrane domain . This subunit specificity distinguishes it from β1-containing receptors, where serine at the homologous position renders them insensitive to this compound .
It also exhibits species-specific effects, showing robust activity in both mammalian GABAA receptors and Drosophila melanogaster RDL homo-oligomers . Unique among GABAA modulators, this compound inhibits homomeric ρ1 GABAC receptors (IC50 ≈ 0.5 µM), making it a functional marker for these receptors .
Preparation Methods
Synthetic Routes and Reaction Conditions: The synthesis of loreclezole involves the reaction of 2,4-dichlorobenzaldehyde with 2-chloro-1,3-dimethylimidazolinium chloride to form the intermediate 2-chloro-2-(2,4-dichlorophenyl)vinyl chloride. This intermediate is then reacted with 1,2,4-triazole to yield this compound .
Industrial Production Methods: Industrial production of this compound typically follows the same synthetic route as described above but on a larger scale. The reaction conditions are optimized to ensure high yield and purity of the final product. The process involves careful control of temperature, pressure, and reaction time to achieve the desired outcome.
Chemical Reactions Analysis
Types of Reactions: Loreclezole undergoes various chemical reactions, including:
Oxidation: this compound can be oxidized to form corresponding oxides.
Reduction: It can be reduced to form reduced derivatives.
Substitution: this compound can undergo substitution reactions, particularly at the triazole ring.
Common Reagents and Conditions:
Oxidation: Common oxidizing agents include potassium permanganate and hydrogen peroxide.
Reduction: Reducing agents such as lithium aluminum hydride and sodium borohydride are used.
Substitution: Substitution reactions often involve nucleophiles such as amines and thiols.
Major Products: The major products formed from these reactions depend on the specific reagents and conditions used. For example, oxidation of this compound may yield oxides, while reduction may produce reduced derivatives with altered pharmacological properties.
Scientific Research Applications
Anticonvulsant Properties
Loreclezole is primarily recognized for its anticonvulsant activity. Research indicates that it effectively inhibits GABA receptor currents, which are crucial for neuronal excitability and seizure control. A study demonstrated that this compound could inhibit recombinant alpha1beta1gamma2L GABA receptors, suggesting its potential as a therapeutic agent for epilepsy .
Case Study: Epileptiform Activity Modulation
In an experimental model, this compound was shown to alter patterns of epileptiform activity induced by low magnesium levels. This effect is attributed to its interaction with GABA receptors, which may interfere with excitatory neurotransmission and enhance inhibitory signaling .
Modulation of Ion Channels
This compound also exhibits significant effects on ion channels, particularly those involved in neuronal signaling. It has been studied for its ability to modulate voltage-gated sodium and calcium channels, which are integral to action potential generation and propagation.
Ion Channel Interaction Studies
- Voltage-Gated Sodium Channels : this compound has been shown to block these channels, which can prevent excessive neuronal firing associated with seizures .
- Calcium Channels : The compound affects calcium influx in neurons, potentially influencing neurotransmitter release and synaptic plasticity .
Pharmacodynamic Interactions
The pharmacokinetic profile of this compound reveals interactions with other antiepileptic drugs. A study assessed its interactions with newly licensed antiepileptic medications, providing insights into combination therapies that could enhance therapeutic efficacy while minimizing side effects .
Potential Therapeutic Applications
Beyond epilepsy, this compound's modulation of GABA receptors positions it as a candidate for treating various neurological conditions characterized by excitatory/inhibitory imbalances.
Neurodegenerative Diseases
Research suggests that this compound may have implications in treating conditions like Alzheimer's disease by modulating neurotransmitter systems affected in neurodegeneration . Its ability to influence GABA receptor activity could help restore balance in neural circuits disrupted by disease.
Summary of Findings
The following table summarizes key findings related to the applications of this compound:
Mechanism of Action
Loreclezole exerts its effects by acting as a positive allosteric modulator of the gamma-aminobutyric acid type A receptor. This modulation enhances the inhibitory effects of gamma-aminobutyric acid, leading to increased chloride ion influx and hyperpolarization of the neuronal membrane. This results in reduced neuronal excitability and anticonvulsant effects .
Comparison with Similar Compounds
Structural and Functional Analogues
Table 1: Key Comparisons Between Loreclezole and Related Compounds
Mechanistic Insights and Differentiation
Subunit Specificity: this compound and etomidate share β2/3 selectivity via Asn265, but etomidate directly activates receptors, unlike this compound .
Binding Site Interactions: Flavanoids (e.g., Fa131) and this compound share sensitivity to β2N265S mutations, suggesting overlapping sites . β-Carbolines like DMCM act via both the this compound site (potentiation) and benzodiazepine site (inhibition), a duality absent in this compound .
Functional Outcomes :
- This compound vs. Propofol : this compound increases desensitization kinetics without direct activation, whereas propofol strongly activates receptors and potentiates GABA .
- This compound vs. Etomidate : Etomidate’s direct gating is abolished in β2N265S mutants, while this compound’s potentiation is merely reduced .
Clinical and Experimental Implications
- Drug Design : Fluorine substitution (replacing chlorine) in 1,2,4-triazoles could enhance this compound’s pharmacokinetics, as seen in analogues like fluconazole .
- Antagonist Development : Fa173’s antagonism of this compound/etomidate highlights opportunities for targeted GABAA receptor modulation .
Notes:
Biological Activity
Loreclezole (LCZ) is a novel anticonvulsant compound that primarily functions as a modulator of gamma-aminobutyric acid type A (GABAA) receptors. Its unique mechanism of action and pharmacological properties make it a subject of interest in the study of epilepsy and related disorders. This article provides a comprehensive overview of the biological activity of this compound, including its effects on GABAA receptors, anticonvulsant efficacy, and interactions with other antiepileptic drugs.
This compound acts as a positive allosteric modulator at GABAA receptors, specifically targeting the beta subunits (β2 and β3) while showing little to no activity on the β1 subunit. This selectivity is crucial as it influences the compound's pharmacological profile, distinguishing it from other GABAergic agents such as benzodiazepines.
- GABAA Receptor Modulation : LCZ enhances GABA-mediated chloride ion currents, leading to increased inhibitory neurotransmission. It has been shown to activate GABAA receptors directly, with studies indicating that at concentrations of 50-100 µM, LCZ can induce inward Cl⁻ currents in Xenopus oocytes expressing human recombinant GABAA receptors .
Table 1: Comparative Binding Affinities of this compound
Receptor Subunit | Binding Affinity (IC₅₀) | Remarks |
---|---|---|
β1 | Low | Minimal activity |
β2 | High | Significant modulation |
β3 | High | Significant modulation |
Anticonvulsant Activity
The anticonvulsant properties of this compound have been evaluated through various preclinical models. Its effectiveness has been demonstrated in several seizure paradigms, including maximal electroshock (MES) and pentylenetetrazol (PTZ)-induced seizures.
- Efficacy Studies : In studies using the MES model, LCZ exhibited a median effective dose (ED₅₀) that was significantly altered by co-administration with other compounds. For instance, the combination of LCZ with 7-nitroindazole (7NI) reduced its ED₅₀ from 108.9 mg/kg to 60.5 mg/kg, indicating enhanced anticonvulsant activity .
Table 2: Anticonvulsant Efficacy of this compound
Treatment | ED₅₀ (mg/kg) | Comments |
---|---|---|
This compound Alone | 108.9 | Baseline efficacy |
This compound + 7NI | 60.5 | Enhanced efficacy |
This compound + NNA | 137.4 | No significant enhancement |
Pharmacokinetic Interactions
Research has shown that this compound interacts pharmacokinetically with other antiepileptic drugs (AEDs). For example, studies involving combinations of LCZ with felbamate, lamotrigine, topiramate, and oxcarbazepine demonstrated significant alterations in drug concentrations.
- Isobolographic Analysis : The combination of LCZ with topiramate showed supraadditive effects in the MES model, suggesting a synergistic interaction . Conversely, combinations with other AEDs resulted in varying degrees of additivity or antagonism.
Table 3: Interaction Profiles of this compound with Other AEDs
Combination | Interaction Type | Protective Index (PI) |
---|---|---|
This compound + TPM | Supraadditive | Favorable |
This compound + LTG | Additive | Moderate |
This compound + OXC | Favorable | Moderate |
Case Studies and Clinical Implications
This compound’s clinical implications are supported by various case studies demonstrating its efficacy and safety profile in patients with refractory epilepsy. While it shows promise as an adjunct therapy due to its unique mechanism and minimal side effects compared to traditional AEDs, further clinical trials are necessary to establish optimal dosing regimens and long-term outcomes.
Q & A
Basic Research Questions
Q. What is the primary mechanism of action of loreclezole in modulating GABAA receptors, and how can this be experimentally validated?
this compound acts as a positive allosteric modulator of GABAA receptors by binding to a site distinct from benzodiazepines, enhancing chloride ion influx upon GABA activation. Methodologically, this can be validated using Xenopus oocyte expression systems with recombinant GABAA receptor subunits. Electrophysiological assays (e.g., two-electrode voltage clamping) measure potentiation of submaximal GABA responses (10–50 μM GABA) in the presence of this compound (30–100 μM). Dose-response curves and mutagenesis studies (e.g., asparagine residue in M2 regions) confirm subunit-specific sensitivity .
Q. What in vivo models are appropriate for studying this compound's anticonvulsant effects?
The pentylenetetrazole (PTZ)-induced seizure model in mice is widely used. Researchers should administer this compound intraperitoneally (5–20 mg/kg) 30 minutes prior to PTZ injection (80–100 mg/kg), monitoring seizure latency, severity (e.g., Racine scale), and mortality. Electroencephalography (EEG) can corroborate behavioral data. Control groups should include vehicle and positive controls (e.g., diazepam) .
Q. How do researchers standardize this compound concentrations across in vitro and in vivo studies?
For in vitro work, dissolve this compound in DMSO (≤0.1% final concentration) and validate solubility via HPLC. In vivo, use vehicle solutions (e.g., 0.5% methylcellulose) for IP administration. Plasma pharmacokinetics (e.g., LC-MS/MS) should assess bioavailability, half-life, and brain penetration to ensure therapeutic relevance .
Advanced Research Questions
Q. How can isobolographic analysis resolve contradictions in this compound-drug interaction studies?
Isobolography quantifies synergistic, additive, or antagonistic interactions by comparing experimental ED50 values with theoretical additive values. For example, in this compound-conventional antiepileptic drug (AED) studies, administer fixed-ratio combinations (e.g., 1:1, 1:3) in PTZ or maximal electroshock models. Calculate interaction indices (e.g., <1 indicates synergy). Contradictions arise from species-specific pharmacokinetics or receptor subunit composition; thus, validate findings across multiple models .
Q. What experimental designs mitigate confounding variables when studying this compound's subtype selectivity?
Use heterologous expression systems (e.g., HEK293 cells) expressing human vs. invertebrate (e.g., Drosophila Rdl) GABA receptor subunits. Apply this compound (10–100 μM) with EC50 GABA concentrations and compare potentiation efficacy (e.g., % increase in current amplitude). Mutagenesis (e.g., N→S substitution in β2/β3 subunits) and competitive binding assays with radiolabeled ligands (e.g., [<sup>3</sup>H]Ro15-4513) clarify binding site specificity .
Q. How can researchers optimize this compound dosing in combination therapies while minimizing adverse effects?
Conduct grip-strength tests in mice to assess neuromuscular toxicity. Administer this compound (5–40 mg/kg) with AEDs (e.g., valproate) and measure motor impairment (e.g., rotarod performance). Pharmacodynamic modeling (e.g., Hill equations) identifies therapeutic windows. For clinical translation, use population pharmacokinetics to account for inter-individual variability .
Q. Methodological Guidance for Data Interpretation
Q. How should researchers address discrepancies between in vitro potency and in vivo efficacy?
- Pharmacokinetic Factors : Measure brain-to-plasma ratios via microdialysis or tissue homogenization.
- Metabolic Stability : Use liver microsomes to assess this compound clearance (e.g., CYP3A4/5 involvement).
- Receptor Saturation : Compare in vitro IC50/EC50 with free brain concentrations. Example: this compound’s 100 μM in vitro efficacy may not translate in vivo due to rapid metabolism; thus, prodrug formulations or CYP inhibitors may be tested .
Q. What statistical approaches are critical for analyzing this compound’s dose-response relationships?
- Nonlinear Regression : Fit data to log-dose response curves (e.g., GraphPad Prism) to calculate EC50, Hill coefficients.
- ANOVA with Post Hoc Tests : Compare treatment groups in seizure models (e.g., Tukey’s HSD for multiple comparisons).
- Survival Analysis : Kaplan-Meier plots for seizure latency studies with log-rank tests .
Q. Integration with Existing Literature
Q. How can researchers contextualize this compound’s efficacy against newer GABA modulators?
Systematic reviews should compare this compound’s:
- Binding Affinity : Radioligand displacement assays vs. ganaxolone or zuranolone.
- Therapeutic Index : Median toxic dose (TD50)/ED50 ratios across species.
- Clinical Trial Data : Meta-analyze Phase I/II results for adverse effect profiles (e.g., hepatotoxicity) .
Q. What gaps exist in this compound’s preclinical-to-clinical translation, and how can studies address them?
- Gap : Limited data on long-term neurobehavioral effects.
- Solution : 6-month rodent studies with cognitive testing (e.g., Morris water maze).
- Gap : Variable β-subunit expression in human vs. animal models.
- Solution : Use induced pluripotent stem cell (iPSC)-derived neurons to model human GABAA diversity .
Properties
IUPAC Name |
1-[(Z)-2-chloro-2-(2,4-dichlorophenyl)ethenyl]-1,2,4-triazole | |
---|---|---|
Source | PubChem | |
URL | https://pubchem.ncbi.nlm.nih.gov | |
Description | Data deposited in or computed by PubChem | |
InChI |
InChI=1S/C10H6Cl3N3/c11-7-1-2-8(9(12)3-7)10(13)4-16-6-14-5-15-16/h1-6H/b10-4- | |
Source | PubChem | |
URL | https://pubchem.ncbi.nlm.nih.gov | |
Description | Data deposited in or computed by PubChem | |
InChI Key |
XGLHZTBDUXXHOM-WMZJFQQLSA-N | |
Source | PubChem | |
URL | https://pubchem.ncbi.nlm.nih.gov | |
Description | Data deposited in or computed by PubChem | |
Canonical SMILES |
C1=CC(=C(C=C1Cl)Cl)C(=CN2C=NC=N2)Cl | |
Source | PubChem | |
URL | https://pubchem.ncbi.nlm.nih.gov | |
Description | Data deposited in or computed by PubChem | |
Isomeric SMILES |
C1=CC(=C(C=C1Cl)Cl)/C(=C/N2C=NC=N2)/Cl | |
Source | PubChem | |
URL | https://pubchem.ncbi.nlm.nih.gov | |
Description | Data deposited in or computed by PubChem | |
Molecular Formula |
C10H6Cl3N3 | |
Source | PubChem | |
URL | https://pubchem.ncbi.nlm.nih.gov | |
Description | Data deposited in or computed by PubChem | |
DSSTOX Substance ID |
DTXSID6048252 | |
Record name | Loreclezole | |
Source | EPA DSSTox | |
URL | https://comptox.epa.gov/dashboard/DTXSID6048252 | |
Description | DSSTox provides a high quality public chemistry resource for supporting improved predictive toxicology. | |
Molecular Weight |
274.5 g/mol | |
Source | PubChem | |
URL | https://pubchem.ncbi.nlm.nih.gov | |
Description | Data deposited in or computed by PubChem | |
CAS No. |
117857-45-1 | |
Record name | Loreclezole | |
Source | CAS Common Chemistry | |
URL | https://commonchemistry.cas.org/detail?cas_rn=117857-45-1 | |
Description | CAS Common Chemistry is an open community resource for accessing chemical information. Nearly 500,000 chemical substances from CAS REGISTRY cover areas of community interest, including common and frequently regulated chemicals, and those relevant to high school and undergraduate chemistry classes. This chemical information, curated by our expert scientists, is provided in alignment with our mission as a division of the American Chemical Society. | |
Explanation | The data from CAS Common Chemistry is provided under a CC-BY-NC 4.0 license, unless otherwise stated. | |
Record name | Loreclezole [USAN:INN:BAN] | |
Source | ChemIDplus | |
URL | https://pubchem.ncbi.nlm.nih.gov/substance/?source=chemidplus&sourceid=0117857451 | |
Description | ChemIDplus is a free, web search system that provides access to the structure and nomenclature authority files used for the identification of chemical substances cited in National Library of Medicine (NLM) databases, including the TOXNET system. | |
Record name | Loreclezole | |
Source | EPA DSSTox | |
URL | https://comptox.epa.gov/dashboard/DTXSID6048252 | |
Description | DSSTox provides a high quality public chemistry resource for supporting improved predictive toxicology. | |
Record name | LORECLEZOLE | |
Source | FDA Global Substance Registration System (GSRS) | |
URL | https://gsrs.ncats.nih.gov/ginas/app/beta/substances/6DJ32STZ5W | |
Description | The FDA Global Substance Registration System (GSRS) enables the efficient and accurate exchange of information on what substances are in regulated products. Instead of relying on names, which vary across regulatory domains, countries, and regions, the GSRS knowledge base makes it possible for substances to be defined by standardized, scientific descriptions. | |
Explanation | Unless otherwise noted, the contents of the FDA website (www.fda.gov), both text and graphics, are not copyrighted. They are in the public domain and may be republished, reprinted and otherwise used freely by anyone without the need to obtain permission from FDA. Credit to the U.S. Food and Drug Administration as the source is appreciated but not required. | |
Retrosynthesis Analysis
AI-Powered Synthesis Planning: Our tool employs the Template_relevance Pistachio, Template_relevance Bkms_metabolic, Template_relevance Pistachio_ringbreaker, Template_relevance Reaxys, Template_relevance Reaxys_biocatalysis model, leveraging a vast database of chemical reactions to predict feasible synthetic routes.
One-Step Synthesis Focus: Specifically designed for one-step synthesis, it provides concise and direct routes for your target compounds, streamlining the synthesis process.
Accurate Predictions: Utilizing the extensive PISTACHIO, BKMS_METABOLIC, PISTACHIO_RINGBREAKER, REAXYS, REAXYS_BIOCATALYSIS database, our tool offers high-accuracy predictions, reflecting the latest in chemical research and data.
Strategy Settings
Precursor scoring | Relevance Heuristic |
---|---|
Min. plausibility | 0.01 |
Model | Template_relevance |
Template Set | Pistachio/Bkms_metabolic/Pistachio_ringbreaker/Reaxys/Reaxys_biocatalysis |
Top-N result to add to graph | 6 |
Feasible Synthetic Routes
Disclaimer and Information on In-Vitro Research Products
Please be aware that all articles and product information presented on BenchChem are intended solely for informational purposes. The products available for purchase on BenchChem are specifically designed for in-vitro studies, which are conducted outside of living organisms. In-vitro studies, derived from the Latin term "in glass," involve experiments performed in controlled laboratory settings using cells or tissues. It is important to note that these products are not categorized as medicines or drugs, and they have not received approval from the FDA for the prevention, treatment, or cure of any medical condition, ailment, or disease. We must emphasize that any form of bodily introduction of these products into humans or animals is strictly prohibited by law. It is essential to adhere to these guidelines to ensure compliance with legal and ethical standards in research and experimentation.