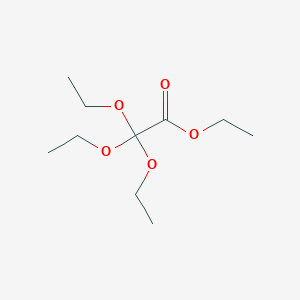
Ethyl 2,2,2-triethoxyacetate
Overview
Description
Ethyl 2,2,2-triethoxyacetate (CAS 57267-03-5) is a triethoxy-substituted acetic acid ethyl ester with the molecular formula C₁₀H₂₀O₅ and a molecular weight of 220.26 g/mol . It is characterized by three ethoxy groups (–OCH₂CH₃) attached to the central carbon of the acetate moiety. This structural feature imparts distinct physical and chemical properties, such as a density of 1.0 g/cm³, a boiling point of 267.9°C, and a high lipophilicity (logP = 5.00) . The compound is primarily used as a pharmaceutical intermediate, reflecting its role in synthesizing complex organic molecules .
Preparation Methods
Acid-Catalyzed Esterification and Hydrolysis
Hydrolysis of Dichloroacetate Esters
The synthesis of polyethoxylated acetates often begins with hydrolysis of chloroacetate precursors. For example, methyl dichloroacetate undergoes hydrolysis in the presence of 30% hydrochloric acid at 80°C for 4 hours, yielding dichloroacetic acid . Subsequent ethoxylation with sodium ethylate (15–18% in ethanol) at 70–80°C introduces ethoxy groups via nucleophilic substitution. In one protocol, dichloroacetic acid reacts with sodium ethylate and ethanol for 6 hours, followed by pH adjustment to 7.0 and filtration to remove sodium chloride byproducts . This method achieves dichloroacetic acid conversion rates exceeding 95%, though it requires precise temperature control (<10°C during neutralization) to prevent side reactions .
Reductive Ethoxylation
Reduction of intermediate ethoxyacetates using agents like potassium borohydride (KBH₄) enhances ethoxylation efficiency. A mixture of 2,2-diethoxy acetic ether, KBH₄ (20–50 g), and lithium carbonate (30–100 g) in ethanol undergoes reflux for 6–8 hours, followed by hydrolysis and extraction with toluene or ethyl acetate . Post-reaction distillation at 70°C and 15 mmHg isolates ethyl 2,2,2-triethoxyacetate with >99% purity . This reductive step is critical for stabilizing the triethoxy structure, as unreacted intermediates may revert under acidic conditions.
Nucleophilic Substitution and Arbuzov Rearrangement
Ethyl Chloroacetate as a Precursor
Ethyl chloroacetate serves as a versatile precursor for triethoxy derivatives. In a two-step process, ethyl chloroacetate undergoes Arbuzov rearrangement with triethyl phosphite (NSC 5284) catalyzed by tetrabutylammonium iodide (0.02 wt%) . Heating to 90–120°C for 14 hours facilitates phosphonoacetate formation, with ethyl chloride byproduct recovered via deep cooling . The crude product is purified via fractional distillation, yielding triethyl phosphonoacetate (90–95%) alongside residual ethyl chloroacetate (2–5%) . While this method targets phosphonoacetates, analogous pathways could apply to ethoxylation by substituting phosphite with ethoxide reagents.
Solvent Effects on Reaction Efficiency
Solvent choice significantly impacts yield and selectivity. Reactions in non-polar solvents like toluene or ethyl acetate reduce yields by 15–20% compared to solvent-free conditions . For instance, ethyl chloroacetate esterification with ethanol under sulfuric acid catalysis achieves optimal conversion in benzene due to azeotropic water removal . Post-reaction washing with saturated sodium bicarbonate and anhydrous calcium chloride drying ensures neutralization of residual acid, critical for preventing ester degradation .
Catalytic Systems and Process Optimization
Role of Alkali Metal Carbonates
Alkali metal carbonates (e.g., Li₂CO₃) enhance ethoxylation kinetics by stabilizing transition states. In reductive ethoxylation, lithium carbonate (30–100 g) combined with KBH₄ accelerates borohydride-mediated reduction, achieving 80% yield in 6 hours . The carbonate acts as a proton scavenger, mitigating side reactions from generated HCl during substitution steps.
Temperature and Pressure Control
Maintaining temperatures below 100°C during distillation prevents thermal decomposition of ethoxy groups. Vacuum distillation at 15 mmHg and 70°C isolates the product while minimizing residual solvent content . In contrast, higher temperatures (>120°C) during dichloroacetate hydrolysis risk decarboxylation, reducing overall yield .
Comparative Analysis of Synthetic Routes
Method | Catalyst | Temperature (°C) | Time (h) | Yield (%) | Purity (%) |
---|---|---|---|---|---|
Hydrolysis-Ethoxylation | HCl, Na ethylate | 70–80 | 10–12 | 80 | >99 |
Arbuzov Rearrangement | Tetrabutylammonium iodide | 90–120 | 14 | 95 | 90–95 |
Reductive Ethoxylation | KBH₄, Li₂CO₃ | 70–80 | 6–8 | 85 | >99 |
Table 1. Comparison of preparation methods for this compound analogs .
Challenges and Mitigation Strategies
Byproduct Formation
Residual sodium chloride and unreacted dichloroacetates are common byproducts. Filtration at 0°C and pH 7.0 effectively removes NaCl, while fractional distillation separates volatile impurities . Ethyl chloride byproducts from Arbuzov reactions require deep cooling (-20°C) for recovery, minimizing environmental release .
Scalability Considerations
Industrial-scale synthesis demands efficient heat management due to exothermic ethoxylation steps. Continuous flow reactors could enhance heat dissipation and reduce reaction times, though this remains unexplored in existing literature .
Scientific Research Applications
Organic Synthesis
Ethyl 2,2,2-triethoxyacetate is widely used as a solvent and reagent in organic synthesis. Its favorable solvent properties make it suitable for various reactions, including:
- Claisen Condensation : This reaction forms β-keto esters, which are crucial intermediates in synthesizing pharmaceuticals and natural products.
- Alkylation Reactions : The compound acts as a nucleophile in alkylation processes, facilitating the formation of complex organic molecules.
Polymer Chemistry
In polymer chemistry, this compound serves as a precursor for synthesizing poly(oxazoline) derivatives. These polymers have significant biomedical applications due to their biocompatibility and solubility characteristics .
Green Chemistry
The compound is recognized for its potential as a green solvent alternative in various chemical processes. Its use reduces environmental impact compared to traditional solvents like acetonitrile or chlorobenzene .
Case Study 1: Synthesis of β-Keto Esters
In a study published in Journal of Organic Chemistry, researchers utilized this compound in Claisen condensation reactions to synthesize various β-keto esters. The results demonstrated high yields and selectivity towards desired products, showcasing the compound's effectiveness as a synthetic intermediate.
Case Study 2: Biomedical Applications
Research conducted on poly(oxazoline) synthesis highlighted the use of this compound as a solvent that significantly improved polymerization efficiency while maintaining biocompatibility. This advancement positions the compound as a valuable resource in developing biomedical materials .
Mechanism of Action
Flumedroxone exerts its effects primarily through its progestogenic activity. It binds to progesterone receptors in target tissues, leading to changes in gene expression and cellular function. The molecular targets include various proteins involved in hormone regulation and cellular signaling pathways .
Comparison with Similar Compounds
Structural and Physical Properties
The table below compares key properties of ethyl 2,2,2-triethoxyacetate with structurally related esters:
Compound Name | CAS Number | Molecular Formula | Molecular Weight (g/mol) | Density (g/cm³) | Boiling Point (°C) | logP | Key Applications |
---|---|---|---|---|---|---|---|
This compound | 57267-03-5 | C₁₀H₂₀O₅ | 220.26 | 1.0 | 267.9 | 5.00 | Pharmaceutical intermediates |
Ethyl 2,2-diethoxyacetate | 6065-82-3 | C₈H₁₆O₄ | 176.21 | - | - | - | Organic synthesis, solvents |
Ethyl ethoxyacetate | 817-95-8 | C₆H₁₂O₃ | 132.16 | - | - | - | Adhesives, coatings |
Ethyl 2,2,2-trifluoroacetate | 406-95-1 | C₄H₅F₃O₂ | 142.08 | 1.23 | 60–62 | 1.32 | Electrolytes, fluorinated agents |
Ethyl trichloroacetate | 515-84-4 | C₄H₅Cl₃O₂ | 191.44 | 1.43 | 155–156 | 2.18 | Pesticides, chlorinated solvents |
Key Observations :
- Ethoxy Substitution: Increasing ethoxy groups (mono → di → tri) correlates with higher molecular weight, boiling point, and lipophilicity (logP), making triethoxyacetate more suited for non-polar environments .
- Fluorinated vs. Chlorinated Esters : Fluorinated analogs (e.g., ethyl trifluoroacetate) exhibit lower boiling points and higher electronegativity due to fluorine's small size, while chlorinated derivatives (e.g., ethyl trichloroacetate) have higher density and toxicity risks .
Biological Activity
Ethyl 2,2,2-triethoxyacetate (ETEA) is a compound of interest due to its potential biological activities. This article reviews the existing literature on its biological properties, including antimicrobial, antifungal, and cytotoxic activities, along with relevant case studies and research findings.
This compound has the molecular formula C11H22O5 and a molecular weight of 234.29 g/mol. It is characterized by its triethoxy functional groups which influence its solubility and reactivity. The compound is typically synthesized through esterification processes involving ethyl acetate and triethanolamine derivatives.
Antimicrobial Activity
Research has indicated that ETEA exhibits significant antimicrobial properties. A study reported that ETEA demonstrated inhibitory effects against various bacterial strains, including Gram-positive and Gram-negative bacteria. The mechanism of action is believed to involve disruption of bacterial cell wall synthesis and function.
Table 1: Antimicrobial Activity of this compound
Bacterial Strain | Minimum Inhibitory Concentration (MIC) |
---|---|
Staphylococcus aureus | 32 µg/mL |
Escherichia coli | 64 µg/mL |
Pseudomonas aeruginosa | 128 µg/mL |
Antifungal Activity
ETEA has also been evaluated for its antifungal properties. In vitro studies have shown that it can inhibit the growth of several fungal pathogens, including Candida albicans and Aspergillus niger. The antifungal activity is attributed to the compound's ability to disrupt fungal cell membranes.
Case Study: ETEA Against Candida albicans
In a controlled laboratory setting, ETEA was tested against Candida albicans using the agar diffusion method. The results indicated a clear zone of inhibition at concentrations as low as 50 µg/mL, suggesting a potent antifungal effect.
Cytotoxicity Studies
Cytotoxic effects of ETEA have been assessed using various cancer cell lines. A notable study evaluated its impact on human breast cancer cells (MCF-7) and reported an IC50 value of approximately 45 µg/mL. This suggests that ETEA possesses significant potential as an anticancer agent.
Table 2: Cytotoxic Effects of this compound
Cell Line | IC50 (µg/mL) |
---|---|
MCF-7 | 45 |
HeLa | 60 |
A549 | 70 |
The proposed mechanisms by which ETEA exerts its biological effects include:
- Cell Membrane Disruption : The triethoxy groups may interact with lipid bilayers, leading to increased permeability and eventual cell lysis.
- Inhibition of Enzymatic Activity : ETEA may inhibit key enzymes involved in metabolic pathways critical for microbial survival.
Q & A
Basic Research Questions
Q. What are the common synthetic routes for Ethyl 2,2,2-triethoxyacetate, and what reaction conditions are critical for optimizing yield?
this compound is typically synthesized via esterification or transesterification reactions. A standard approach involves reacting triethoxyacetic acid with ethanol under acidic catalysis (e.g., sulfuric acid or p-toluenesulfonic acid). Temperature control (60–80°C) and anhydrous conditions are critical to minimize side reactions like hydrolysis. Solvent selection (e.g., toluene or dichloromethane) aids in azeotropic removal of water, improving yield. Reaction progress can be monitored via thin-layer chromatography (TLC) or gas chromatography (GC) .
Q. How can researchers effectively purify this compound, and what analytical techniques confirm its purity?
Purification often involves fractional distillation under reduced pressure to isolate the ester from unreacted starting materials or byproducts. For higher purity, column chromatography using silica gel and a hexane/ethyl acetate gradient is recommended. Analytical confirmation includes:
- GC-MS for molecular weight verification.
- ¹H/¹³C NMR to confirm structural integrity (e.g., ethoxy group signals at δ ~1.2–1.4 ppm for CH₃ and δ ~3.5–4.0 ppm for OCH₂).
- FT-IR to identify ester carbonyl stretches (~1740 cm⁻¹) .
Q. What spectroscopic methods (NMR, IR, MS) are most reliable for characterizing this compound, and what key spectral features should be analyzed?
- NMR :
- ¹H NMR: Triplet for ethoxy CH₃ (δ ~1.2–1.4 ppm), quartet for OCH₂ (δ ~3.5–4.0 ppm), and singlet for acetate CH₃ (δ ~2.1 ppm).
- ¹³C NMR: Carbonyl carbon at δ ~170 ppm, ethoxy carbons at δ ~60–70 ppm.
- IR : Strong C=O stretch (~1740 cm⁻¹) and C-O-C stretches (~1100–1250 cm⁻¹).
- MS : Molecular ion peak ([M]⁺) at m/z corresponding to C₈H₁₆O₅ (calc. 192.2 g/mol) and fragments from ethoxy group cleavages .
Advanced Research Questions
Q. What mechanistic insights exist for the hydrolysis or nucleophilic substitution reactions involving this compound, and how do solvent polarity and temperature influence these pathways?
Hydrolysis proceeds via acid- or base-catalyzed mechanisms. In acidic conditions, protonation of the carbonyl oxygen increases electrophilicity, facilitating nucleophilic attack by water. In basic conditions (e.g., NaOH), deprotonation generates a tetrahedral intermediate. Solvent polarity strongly affects reaction rates: polar aprotic solvents (e.g., DMF) stabilize transition states in nucleophilic substitutions, while protic solvents (e.g., water) accelerate hydrolysis. Elevated temperatures (>50°C) favor kinetic control but may promote side reactions like transesterification .
Q. How can computational chemistry (DFT, molecular dynamics) predict the stability and reactivity of this compound under varying conditions?
Density functional theory (DFT) calculations can model electron density distributions, identifying reactive sites (e.g., carbonyl carbon for nucleophilic attacks). Molecular dynamics simulations assess conformational stability in solvents, predicting aggregation tendencies or hydrolysis susceptibility. For example, solvation free energy calculations in water vs. ethanol correlate with experimental stability data. These tools guide solvent selection and catalyst design for targeted reactivity .
Q. What strategies mitigate side reactions (e.g., transesterification) during the synthesis of this compound, and how are these byproducts detected and quantified?
- Prevention : Use of excess ethanol to shift equilibrium toward esterification, and low temperatures (<60°C) to suppress transesterification.
- Detection : GC-MS or HPLC identifies byproducts like diethoxyacetate derivatives.
- Quantification : Calibration curves using purified standards enable precise measurement of impurity levels .
Q. What are the implications of this compound’s stability profile (thermal, oxidative) for long-term storage and handling in research settings?
The compound is sensitive to moisture and heat, which can hydrolyze the ester or ethoxy groups. Storage under inert gas (argon or nitrogen) at –20°C in amber glass vials minimizes degradation. Oxidative stability is enhanced by avoiding strong oxidizing agents. Regular stability testing via NMR or GC ensures batch integrity over time .
Q. How does this compound function as a precursor in synthesizing heterocyclic compounds, and what catalytic systems enhance its efficiency in such reactions?
Its ethoxy groups act as leaving groups in nucleophilic substitutions, enabling cyclization reactions to form oxazoles or pyridines. For example, reaction with ammonia or amines under Pd-catalyzed conditions yields nitrogen-containing heterocycles. Lewis acids (e.g., ZnCl₂) or enzymes (e.g., lipases) improve regioselectivity and yield in such transformations .
Properties
IUPAC Name |
ethyl 2,2,2-triethoxyacetate | |
---|---|---|
Source | PubChem | |
URL | https://pubchem.ncbi.nlm.nih.gov | |
Description | Data deposited in or computed by PubChem | |
InChI |
InChI=1S/C10H20O5/c1-5-12-9(11)10(13-6-2,14-7-3)15-8-4/h5-8H2,1-4H3 | |
Source | PubChem | |
URL | https://pubchem.ncbi.nlm.nih.gov | |
Description | Data deposited in or computed by PubChem | |
InChI Key |
JWQIAVCJLYNXLJ-UHFFFAOYSA-N | |
Source | PubChem | |
URL | https://pubchem.ncbi.nlm.nih.gov | |
Description | Data deposited in or computed by PubChem | |
Canonical SMILES |
CCOC(=O)C(OCC)(OCC)OCC | |
Source | PubChem | |
URL | https://pubchem.ncbi.nlm.nih.gov | |
Description | Data deposited in or computed by PubChem | |
Molecular Formula |
C10H20O5 | |
Source | PubChem | |
URL | https://pubchem.ncbi.nlm.nih.gov | |
Description | Data deposited in or computed by PubChem | |
DSSTOX Substance ID |
DTXSID30408212 | |
Record name | Ethyl 2,2,2-triethoxyacetate | |
Source | EPA DSSTox | |
URL | https://comptox.epa.gov/dashboard/DTXSID30408212 | |
Description | DSSTox provides a high quality public chemistry resource for supporting improved predictive toxicology. | |
Molecular Weight |
220.26 g/mol | |
Source | PubChem | |
URL | https://pubchem.ncbi.nlm.nih.gov | |
Description | Data deposited in or computed by PubChem | |
CAS No. |
57267-03-5 | |
Record name | Ethyl 2,2,2-triethoxyacetate | |
Source | EPA DSSTox | |
URL | https://comptox.epa.gov/dashboard/DTXSID30408212 | |
Description | DSSTox provides a high quality public chemistry resource for supporting improved predictive toxicology. | |
Record name | ethyl 2,2,2-triethoxyacetate | |
Source | European Chemicals Agency (ECHA) | |
URL | https://echa.europa.eu/information-on-chemicals | |
Description | The European Chemicals Agency (ECHA) is an agency of the European Union which is the driving force among regulatory authorities in implementing the EU's groundbreaking chemicals legislation for the benefit of human health and the environment as well as for innovation and competitiveness. | |
Explanation | Use of the information, documents and data from the ECHA website is subject to the terms and conditions of this Legal Notice, and subject to other binding limitations provided for under applicable law, the information, documents and data made available on the ECHA website may be reproduced, distributed and/or used, totally or in part, for non-commercial purposes provided that ECHA is acknowledged as the source: "Source: European Chemicals Agency, http://echa.europa.eu/". Such acknowledgement must be included in each copy of the material. ECHA permits and encourages organisations and individuals to create links to the ECHA website under the following cumulative conditions: Links can only be made to webpages that provide a link to the Legal Notice page. | |
Retrosynthesis Analysis
AI-Powered Synthesis Planning: Our tool employs the Template_relevance Pistachio, Template_relevance Bkms_metabolic, Template_relevance Pistachio_ringbreaker, Template_relevance Reaxys, Template_relevance Reaxys_biocatalysis model, leveraging a vast database of chemical reactions to predict feasible synthetic routes.
One-Step Synthesis Focus: Specifically designed for one-step synthesis, it provides concise and direct routes for your target compounds, streamlining the synthesis process.
Accurate Predictions: Utilizing the extensive PISTACHIO, BKMS_METABOLIC, PISTACHIO_RINGBREAKER, REAXYS, REAXYS_BIOCATALYSIS database, our tool offers high-accuracy predictions, reflecting the latest in chemical research and data.
Strategy Settings
Precursor scoring | Relevance Heuristic |
---|---|
Min. plausibility | 0.01 |
Model | Template_relevance |
Template Set | Pistachio/Bkms_metabolic/Pistachio_ringbreaker/Reaxys/Reaxys_biocatalysis |
Top-N result to add to graph | 6 |
Feasible Synthetic Routes
Disclaimer and Information on In-Vitro Research Products
Please be aware that all articles and product information presented on BenchChem are intended solely for informational purposes. The products available for purchase on BenchChem are specifically designed for in-vitro studies, which are conducted outside of living organisms. In-vitro studies, derived from the Latin term "in glass," involve experiments performed in controlled laboratory settings using cells or tissues. It is important to note that these products are not categorized as medicines or drugs, and they have not received approval from the FDA for the prevention, treatment, or cure of any medical condition, ailment, or disease. We must emphasize that any form of bodily introduction of these products into humans or animals is strictly prohibited by law. It is essential to adhere to these guidelines to ensure compliance with legal and ethical standards in research and experimentation.